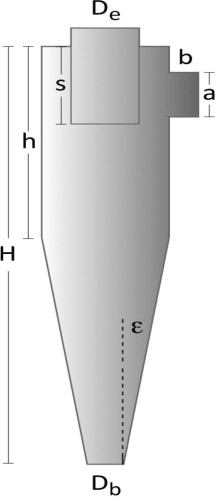
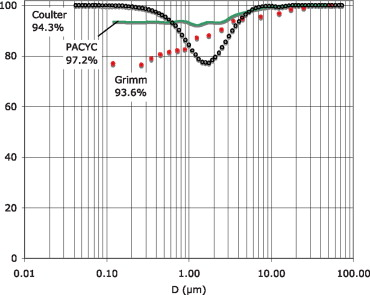
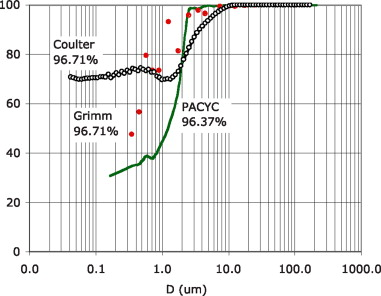
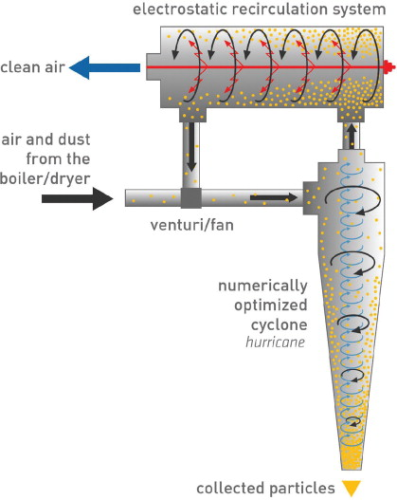
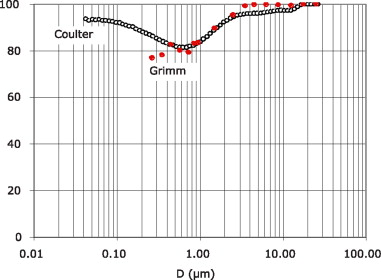
![Figure 6. Grade efficiencies for varying concentrations. (MVD=12.5 µm, ?global subset of [97.23, 98.96] %)](/media/2bzkccng/79601a59-309b-4476-b9b6-095996f3323b.jpeg)
A pdf version of this article is available on the right-hand side under 'Downloads'.
The optimised design of gas cyclones is of particular relevance for the recovery of highly valuable products, such as in the production of active pharmaceutical ingredients (API) through drying systems such as spray or fluid bed drying[1]. Traditionally, these have been captured with reverse flow gas cyclones, but fine and low density particles remain difficult to capture[2]. Bagfilters, although highly efficient, are to be avoided due to possible contamination problems and filter product hold-up.
In addition, small electrostatic precipitators are difficult to clean, and consequently have not been used for this purpose. This article addresses the use of optimised designs of reverse-flow gas cyclones (Hurricanes) that can be coupled with mechanical/electrostatic recirculation systems (ReCyclone® systems). The Hurricane designs are the solution of numerical global optimisation problems, with the objective of maximising collection efficiency, while obeying several imposed operating and geometrical constraints[3]. The simulation model used by the optimiser is based on the predictive properties of a finite diffusivity model[4]. Recently, this model was extended to deal with recirculation[5], allowing the possibility of optimising the already highly efficient ReCyclone systems. Both the Hurricane cyclones and the ReCyclone systems can be made with a break-apart construction, making them compliant with the needs of the API industries.
Cyclones are gas-solid separation devices characterised by low investment and operating costs, which have been used in the API industries for valuable product recovery[2]. The simulation of reverse-flow cyclones has been the subject of several different approaches[6], but none of the proposed theories is capable of consistently giving good predictions when applied to predicting experimental grade-collections obtained with different geometries, operating conditions and particle-size distributions. Of all these, the Mothes and Loffler[4] model gives predictions that are better correlated with available data[[6], [7] and [8]]. However, the model predictions are dependent on the knowledge of the particles turbulent dispersion coefficient, which depends on operating conditions, cyclone geometry and particle size. These difficulties have led cyclone designers to base their geometries on empirical testing, such that a widely accepted basic rule to succeed in cyclone design is to use only geometries that have been experimentally tested[9].
Since each cyclone manufacturer has its own high efficiency (HE) design, the question of which design is indeed better for a particular application is relevant. Also, it is highly unlikely that the optimum design can be found by empirical testing, as there are too many design parameters involved. This has been experimentally confirmed by Maa et al.[2], who tried to improve spray-dryer recovery using re-designed cyclones. Despite various attempts at re-designing the cyclones, no improvement could be observed for capturing particles below about 2 μm, and severe losses (up to 20%) were observed.
In a previous paper[3] optimisation problems were formulated for maximising cyclone collection and a ratio of cost effectiveness. The problems included several constraints on geometry, pressure drop and saltation velocity, thus ensuring that feasible cyclones with efficiencies near the design efficiency could be obtained. The solution to one of these problems (the Hurricane cyclones) was shown at laboratory, pilot and full-scale tests to be superior to other high-efficiency designs available in the market and in the scientific literature[[3], [5], [10] and [11]].
Recognising that particle physical properties (size, specific gravity, porosity) and concentration play a very important role in particle capture in cyclones, a computer program (PACYC – Particle Agglomeration in CYClones) was recently developed, coupling the agglomeration model of Ho and Sommerfeld[12] with the Mothes and Loffler[4] or the Salcedo et al.[5] model of particle capture, respectively for single cyclones and for ReCyclone systems.
Hurricane cyclones
The numerical optimisation of a reverse-flow cyclone can be posed as the need to maximise any appropriate ‘profit' function. This is subject to equality constraints, including relevant design equations (cyclone modelling theory), particle size distribution and particle density, gas flow rate and dust load, gas temperature, and density and viscosity. In addition, there are inequality constraints, including pressure loss, saltation velocity and various geometric constraints.
One of the possible profit functions is global collection efficiency. The equality constraints represent the problem data and the cyclone modelling theory. On the other hand, the inequality constraints represent bounds on the decision variables (the eight cyclone dimensions) and eventually on several dependent variables. Based on available literature, the following set of constraints was employed:
(1) P<1500 Pa
(2) 6.8°< ε <16°
(3) 0.5<4ab/π De2<0.735
(4) uin/us<1.25
The first constraint limits the cyclone pressure drop, since this is directly related to operating costs. The second constraint limits the cyclone cone semi-angle, and reflects values that are associated with an easy dislodgement of the collected dust along the cyclone cone walls. The third constraint simply states that the effective cyclone gas discharge area should be larger than the entry section, and the bounds were found by inspecting sound cyclone designs. The last constraint puts an upper limit to the cyclone mean entry velocity, but only by comparison with the saltation velocity, which is a function of cyclone geometry (variable during the optimization process), particle density and operating conditions.
The geometric constraints were as follows:
(5) s
(6) 0.5Debe
(7) 0.5(D-De)>b
(8) s>1.25a
(9) 2.3De(D2/ab)1/3
Condition (7) allows for an easy transition at the cyclone entry, while condition (8) avoids short-circuiting of the gas. The last constraint reflects that the natural length of the cyclone (the length of the descending vortex below the vortex finder) must not exceed the available cyclone height.
The proposed problems are non-convex non-linear constrained optimisation problems, with eight degrees of freedom, since the best diameter for a given set of operating conditions is not known. These problems can be solved using a global optimiser[13]. A Hurricane cyclone is the main output from a particular cyclone design optimisation problem[14], but other problems may be formulated for any particular situation, eventually producing different cyclone optimal designs.
Figure 2 shows the expected grade-efficiencies for a fine pharmaceutical powder (MVD=5.5 μm, ρp=1680 kg/m3, Cin=3.4 g/m3), for a 530 mm Hurricane operating at 200-250 mm w.g. The Coulter and Grimm predictions were derived from experimental runs with other powders, on a 450 mm pilot Hurricane, and corrected for particle density, concentration and operating conditions[15]. The PACYC predictions were directly derived for the 530 mm Hurricane operating with the particular powder in question. The experimental grade-efficiencies from this powder are not available, but the global efficiency varied from ≈ 96-98%, in very close agreement with the PACYC predictions.
Figure 3 shows the experimental grade and global collection obtained in a pilot 450 mm Hurricane, for a food ingredient powder (powder (MVD=13.6 μm, ρp=1608 kg/m3, Cin=0.64 g/m3).
The Hurricane's high efficiency for particles smaller than about 1 μm is evident. Although the PACYC model does not follow the particles' behaviour for the entire size spectrum, it clearly captures the high collection observed for fine particles, thus allowing a better prediction of the grade and global efficiencies.
Recyclone® systems
Figure 4 shows a recirculation loop, comprising one reverse-flow cyclone (collector) upstream from a recirculation cyclone (concentrator). These systems are inherently more efficient than single cyclones, or than cyclones in series, for a comparable pressure drop, and have been patented[[14] and [16]]. The recirculator itself may be simply a mechanical straight-through cyclone or it may have an electric field superimposed, to increase recirculation of fine particles to the cyclone collector. The gas may be recirculated by an appropriate fan or by a venturi. Since venturis are easily cleaned, they should be adequate for recirculation within API pharmaceutical industries.
ReCyclone systems using only centrifugal forces in the recirculator (mechanical ReCyclones) have been extensively tested at laboratory, pilot and industrial scales[[5], [10] and [11]]. Recently, the adoption of electrostatic recirculation has brought these systems to a higher level for fine particle collection, even at very low concentrations (< 0.1g/m3). Figure 5 shows the experimental grade efficiencies as measured on-line by a Grimm 1.108 laser sizer and off-line by a Couter LS230. The agreement is remarkable, showing very high collections even for sub-micrometer particles.
Figure 6 shows the effect of increased concentration in shifting the grade-efficiency curves towards higher values, for a powder with MMV=12.5μm. Once more, collection for very fine particles is very large.
Although not shown here, experiments at pilot-scale show that electrostatic ReCyclones can capture dust as fine as 0.3-0.5 μm median-volume with efficiencies as high as 85-90%. Electrostatic ReCyclones use about 1/10th to 1/20th of the high voltage power typical of electrostatic precipitators, are immune to dust resistivity (since dust is not captured on the recirculator walls but only on the cyclone collector, which is not electrified) and may be built with a break-apart configuration, for easier cleaning and to minimise contamination. Roughly, and at equivalent pressure losses, electrostatic ReCyclones can reduce emissions from Hurricanes by as much as 75-80%, and from other HE cyclones, by more than 90%.
Conclusions
Numerically optimised cyclones (Hurricanes) and recirculation systems have been shown to be highly efficient for the capture of fine particles, such as usually occurring in food ingredients and pharmaceutical API production. Numerically optimised cyclones and ReCyclone systems were designed by solving appropriate optimisation problems.
The abnormal high collection of fine particles often observed with these systems is attributed to particle agglomeration within the turbulent cyclone flow field. The PACYC model was developed based on the Ho and Sommerfeld[12] particle agglomeration model, superimposed on the Mothes and Loffler[4] particle collection model. Recent developments couple the agglomeration model with the Salcedo et at.[5] model for particle capture in recirculation systems.
On average, Hurricanes can reduce emission of comparable pressure drop high efficiency cyclones by about 40-60%, mechanical ReCyclones by about 75-80% and electrostatic ReCyclones by more than 90%. These systems may be built on a break-apart configuration, for easier cleaning and to avoid unwanted contamination, and their effectiveness has already been shown at pilot and industrial scales, for a variety of very fine dusts.
The PACYC model is being currently extended to deal with mechanical and electrostatic recirculation, and ongoing research is being carried out to improve the rate of recirculation using venturi devices, to promote adequate recirculation without contamination concerns.
References
1 S.A. Shoyele and S. Cawthorne, Particle engineering techniques for inhaled biopharmaceuticals, Advanced Drug Delievery Reviews 58 (9–10) (2006), pp. 1009–1029. Article | PDF (538 K) | View Record in Scopus | Cited By in Scopus (30)
2 Y.-F. Maa, P.A. Nguyen, P.-A.K. Sit and C.C. Hsu, Spray-Drying Performance of a Bench-Top Spray Dryer for Protein Aerosol Powder Preparation, Biotechnology and Bioengineering 60 (3) (1998), pp. 301–309. Full Text via CrossRef | View Record in Scopus | Cited By in Scopus (30)
3 R.L. Salcedo and M.G. Cândido, Global optimization of reverse-flow gas-cyclones: application to small-scale cyclone design, Separation Sci. and Technology 36 (12) (2001), p. 2707. Full Text via CrossRef | View Record in Scopus | Cited By in Scopus (4)
4 H. Mothes and F. Loffler, Prediction of Particle Removal in Cyclone Separators, Int. Chem. Eng. 28 (1988), pp. 231–240 Paiva, J., R. Salcedo and P. Araujo, 2009, Particle Agglomeration in Cyclones: PACYC Model, World ChemEngCong'09.. View Record in Scopus | Cited By in Scopus (45)
5 R.L.R. Salcedo, A.M. Fonseca, V.G. Chibante and G. Cândido, Fine Particle Capture in Biomass Boilers with Recirculating Gas Cyclones: theory and practice, Powder Technology 172 (2007), pp. 89–98. Article | PDF (1383 K) | View Record in Scopus | Cited By in Scopus (2)
6 R.L. Salcedo and A.M. Fonseca, Grade-efficiencies and particle size distributions from sampling cyclones. In: P. Cheremisinoff, Editor, Mixed-Flow Hydrodynamics, Gulf Publishers (1996), pp. 539–561 Ch. 23.
7 M. Bohnet and T. Lorenz, Separation efficiency and pressure drop of cyclones at high temperatures. In: R. Clift and J.P.K. Seville, Editors, Gas Cleaning at High Temperatures, Blackie Academic and Professional (1993), pp. 17–31.
8 R. Clift, M. Ghadiri and A.C. Hoffman, A Critique of Two Models for Cyclone Performance, AIChE J 37 (1991), pp. 285–289. Full Text via CrossRef | View Record in Scopus | Cited By in Scopus (34)
9 W.L. Heumann, Cyclone Separators: a family affair, Chem. Eng. June (1991), pp. 118–123.
10 R.L. Salcedo and M.J. Pinho, Pilot and Industrial-Scale Experimental Investigation of Numerically Optimized Cyclones, Ind. Eng. Chem. Res. 42 (1) (2002), p. 145.
11 R.L. Salcedo, V.G. Chibante and I. Sôro, Laboratory, pilot and industrial-scale validation of numerically optimized reverse-flow gas cyclones, Trans. of the Filt. Soc. 4 (3) (2004), p. 220.
12 C.A. Ho and M. Sommerfeld, Modelling of micro-particle agglomeration in turbulent flows, Chem. Eng. Sci. 57 (2002), p. 3073.
13 R. Salcedo, Solving Non-Convex NLP and MINLP Problems with Adaptive Random-Search, Ind. Eng. Chem. Res. 31 (1) (1992), pp. 262–273. Full Text via CrossRef | View Record in Scopus | Cited By in Scopus (47)
14 Salcedo, R.L., Recirculation cyclones for dedusting and dry gas cleaning, WO0141934, 14.06.2001.
15 W.L. Heumann, Industrial Air Pollution Control Systems, McGraw-Hill (1997).
16 Salcedo, R.L., Process of electrostatic recirculation for dedusting and gas cleaning and device thereof, WO20088147233, 04.11.2008.