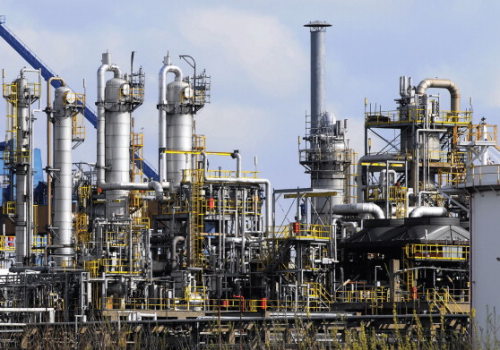
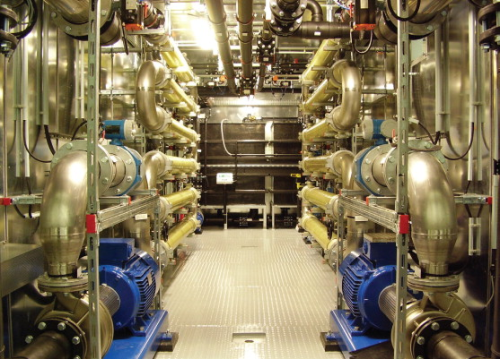
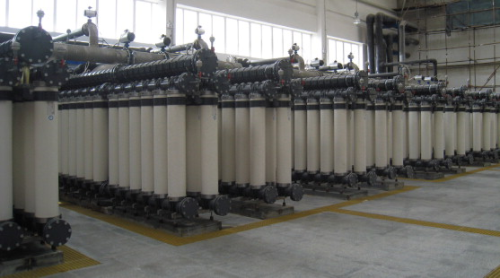
Fine chemicals are chemicals produced in small-to-medium quantities but their definition is imprecise and wide ranging, including pharmaceuticals. Here we concentrate on agrichemicals, specialist chemicals and high purity chemicals typically synthesised in small batches for producing products such as dyes, pigments, coatings, flavors, fragrances, lubricants and microelectronic grade chemicals.
Introduction
In an issue of Filtration+Separation, published in July/August 2008, Ken Sutherland outlined the range of products produced in the chemicals industry. He focused on bulk chemicals but gave a useful summary of the chemicals industry as a whole, including pharmaceuticals and biotechnical products. This represented the largest sector of the membrane market in 2007, with 30.6% of the global total. The fine chemicals sector represents around a quarter of the whole membranes chemical market. This smaller sector is characterised by a range of diverse applications for membrane technology, covering three main aspects:
• production of process water, subsequently utilised in the manufacturing process or in the dilution of fine chemicals;
• filtration and separation of the fine chemicals themselves; and
• treatment of effluent from fine chemicals production processes.
Fine chemicals are pure, single substances that are typically produced by chemical reactions for highly specialised applications. The fine chemicals produced can be categorised into active pharmaceutical ingredients and their intermediates, agrichemicals, speciality chemicals and high-purity chemicals for technical applications.
In contrast to bulk chemicals, which are produced in massive quantities by standardised reactions for subsequent direct use, fine chemicals are custom-produced in smaller quantities for special uses. The methods of production need to be flexible, and owing to the relatively small volumes required and the diversity of types, the definition of fine chemicals is wide ranging. Production is more expensive than for bulk chemicals, generates more effluent that can be difficult to treat, and requires a higher research investment per unit weight produced. Fine chemicals are, however, produced in industrial quantities unlike research chemicals, but batch production tends to be common as opposed to continuous production for bulk chemicals.
Excluding pharmaceutical chemicals and their intermediaries, the remaining types of fine chemicals covered here include:
Agrichemicals
These mainly include biocides, pesticides, herbicides and other specialised chemicals that are used in agriculture to inhibit or kill pests and weeds and improve crop yields.
Specialist chemicals
These are a diverse category produced for a range of technical applications, and are generally sold based on the performance characteristics achieved rather than price per unit weight. Types include:
• inks, paints and other coatings;
• performance-enhancing additives;
• photographic chemicals;
• soap, detergents, toiletries and perfumes;
• explosives;
• glues; and
• essential oils.
High purity chemicals
This category includes especially pure, high quality chemicals with low or undetectable levels of contaminants. Examples include specialist solvent and aqueous formulations for microelectronics. High purity chemicals are produced in batches and typically membrane technology is employed both at the high purity processing site and also to filter out impurities at the end-user site prior to use. These chemicals are compositionally less complex than agrichemicals or specialist chemicals but they are included in this summary because they must be significantly more pure from the perspective of dissolved and particulate contaminants.
Process water production
The types of process water required for the production or dilution of agrochemicals, speciality chemicals and high purity chemicals can vary considerably due to the vast range of fine chemicals available and their production processes. Specifications for process water are typically defined in terms of various parameters, including resistivity, microorganism content, pyrogen (endotoxins) levels, reactive silica levels and total organic carbon (TOC) levels. In fine chemical processing typically Purified Water, Apyrogenic Water and High Purity Water is required dependent on the process employed. These types of process water are defined in the table below.
Purified water has a resistivity greater than 0.2 Megohm.cm (at 25°C) but is defined primarily as having a microorganisms content of less than 100 CFU/ml as well as a total organic carbon (TOC) level of <0.5 mg/l as C. Apyrogenic water is purer still at greater than 0.8 Megohm.cm but a maximum level of 0.35 EU/ml pyrogens (endotoxins) is the main determinant specification for that grade of water. High purity water has a resistivity of at least 10 Megohm.cm.
Membrane technology has a significant part to play in the production of these grades of process water for use in fine chemicals production. Depending on the feed water available, additional membrane technologies may be incorporated as pre-treatment technologies. Ultrafiltration (UF) or microfiltration (MF) technology can be effectively used as a pre-treatment for reverse osmosis (RO) depending on the nature and variability of the feed supply to the water treatment system.
UF and MF technology can also be used for final polishing of pure water and removal of bacterial endotoxins, bacteria and particles.
With a resistivity of 0.2 Megohm.cm, purified water can be produced using single pass RO systems followed by the use of ultraviolet (UV) emission technology to meet the requirement for low total organic carbon (TOC) levels.
RO systems would be fitted with high rejection (HR) membranes. RO is a cross-flow membrane separation process providing a level of filtration down to ionic levels for removal of dissolved salts. Permeate is produced from the membrane with the majority of the dissolved content of the feed transferred to the waste concentrate stream. Depending on the quality of the feed water supply, RO membranes can be configured in an array system to give an overall concentrate stream flow as low as 10% of the feed supply, equating to an overall system recovery of up to 90%. The salts present in the feed supply are then concentrated in the rejected concentrate stream, which must then be treated by subsequent processes on-site or discharged to the sewerage system for further treatment by the local wastewater treatment company.
Individual RO membranes typically reject 95–98 % of the total dissolved solids (TDS) in the feed supply, thereby reducing the ionic loading onto downstream processes. HR membranes reject at the higher end of this range.
Either electro deionisation (EDI), utilising membranes, or ion exchange technologies are required for producing high purity water; both technologies utilise ion exchange resins. EDI is a continuous compact process that eliminates the requirement for regeneration chemicals and waste neutralisation that are synonymous with conventional ion exchange systems. However, the process uses electricity, with the associated cost. EDI is mainly used for deionisation downstream of RO for polishing and removal of silica and other ions. When processing RO permeate, EDI systems typically achieve better than 99.5% salt rejection and they can produce up to 18 Megohm.cm resistivity.
Feedwater entering an EDI system flows through membrane compartments containing ion exchange resin. An electric potential drives the passage of cations through cation-permeable membranes and anions through anion-permeable membranes into a waste stream. Pure water remains in the compartment and leaves the system as the treated water stream. An example of an EDI system is Veolia's short cycle RAPIDE Strata system.
The various types of process water described are utilised throughout the fine chemicals industry.
Process water type | Resistivity - Megohm.cm at 25ºC | Maximum microorganisms - CFU/ml | Maximum Total Dissolved Solids (TDS) - mg/l | Pyrogens (Endotoxins) - EU/ml | Reactive silica - µg/l as SiO2 | Total Organic Carbon (TOC) - mg/l as C |
Purified | 0.2 | 100 | 1 | Not specified | 100 | <0.5 |
Apyrogenic | 0.8 | 0.1 | 1 | 0.25 | 100 | 0.05-0.07 |
High purity | 10 | 1 | 0.5 | Not specified | 20 | Not specified |
Direct filtration and separation
An area of application for polytetrafluoroethylene (PTFE) membranes is in the filtration of high purity chemicals. We asked Stephen Lux, a product specialist at W. L. Gore and Associates, to outline one of his company's areas of focus – filters for the production of high purity chemicals. He told us that purity levels required in microelectronics, particularly the semiconductor industry, “…are tremendously beyond those provided by the manufacturer of the commodity chemical subsequent to the reaction process.”
Chemicals are purified in small to medium-scale facilities utilising membrane technology. Batch sizes are typically 1,000–10,000 litres. Contaminants such as trace metals must be reduced to the ppb or ppt level. Lux added: “Particulate contamination – the focus of our technology – would typically be no more than tens or hundreds of particles per mL, each particle typically no larger than 50–100 nm. This is roughly equivalent to one grain of salt in a football stadium.”
A US high-purity chemical producer was preparing for the manufacture of a new semiconductor-grade solvent stripper. Due to capital funding restraints, the solvent stripper had to be processed on the manufacturer's existing equipment. The new product required a tighter particle count specification in a shorter time than the existing filter could provide. The initial particle count was estimated at >5,000,000 Counts/mL at 0.2 μm particle size.
A batch process was used on site to recirculate the solvent stripper until the particle count was reduced to <250 Counts/mL. The original process utilised 0.05 μm rated cartridge filters and took an average of 40 minutes to clean up with filter replacement required after every batch.
The 0.05 μm cartridges were replaced in the existing system with Gore 0.04 μm PTFE membrane cartridges, which had both higher particle retention and a higher flow rate. Lux commented: “The clean up time was reduced from an average of forty minutes to less than three minutes, and three batches were processed before filter change-out was required.” Gore says that the number of filters required was reduced by two-thirds, reducing the filtration cost, and throughput increased without capital expense or increased labour cost.
Gore's PTFE membrane is manufactured in a flat sheet and is pleated to form standard cartridge-sized configurations. Both hydrophobic and hydrophilic PTFE membranes are available depending on the types of high purity chemicals to be filtered.
Rather than membranes, use of which is best suited to the sub-micron requirements of high purity chemicals, specialist absolute rated media cartridge filters are employed extensively across other fine chemicals sectors. Examples include the high flow microfibre media cartridges manufactured by Velcon Filters. These are used for filtering coatings, adhesives, and their intermediates such as diisocyanate. The high flow filters fit into bag filter housings and are used by a range of fine chemicals producers such as BASF.
Effluent treatment
The most extensive application of membrane technology is in the treatment of effluent from processes where fine chemicals are produced. Companies like Wehrle Environmental and inge watertechnologies design and build process systems for the treatment of fine chemicals effluent for subsequent treatment by wastewater treatment companies, discharge to local watercourses, or for re-use on site to use as an alternative supply of process water, with the benefits of minimizing raw water supply and effluent discharge costs.
Wehrle has a number of reference sites where highly organic-loaded waste streams from Fine Chemical producers have been treated using the Biomembrat membrane bioreactor (MBR) system. For example, 100 m3/day of effluent from the production of textile auxiliaries (washing powder, softeners and finishings) and from the production of preservatives for paint and dyes is treated at a Thor Chemie site. The chemical oxygen demand (COD) of the fine chemicals wastewater is typically 6,000–7,000 mg/l, but with a high component of biologically degradable organic contamination.
The key to effective wastewater treatment by MBR is selection and design of the correct membrane and bioreactor for each specific application. Effluent initially enters a bioreactor tank where microorganisms feed on the wastewater aerobically in a complex ecosystem. This reduces organics through microbial respiration and assimilation processes. Oxygen required for the biological process is provided by blowers which supply air to venturi jet aerators installed inside the bioreactor. The dissolved oxygen in the wastewater is continuously monitored and automatic adjustments are made to the blowers. A biomass concentration of more than four times the value of typical conventional activated sludge plant is reached in MBR bioreactors. Some of the waste sludge is periodically removed from the bioreactor to maintain the desired concentration.
Separation is undertaken by Wehrle within a highly efficient UF system that retains solids and molecules larger than 0.02 μm and is typically installed in a container (see Figure 2) alongside the bioreactor tank. Recirculated effluent enters a stainless steel header system feeding dedicated recirculation pumps and membrane modules arranged in series. Each UF module contains in excess of 350×8mm polymeric membrane tubes. Effluent is pumped along the membrane surface at high velocity ensuring adequate turbulence, minimising membrane fouling and enhancing cleaning efficiency. Permeate is removed through the membrane wall, which provides an absolute barrier, producing treated effluent free from bacteria and suspended solids.
In the textile industry, large quantities of water are used in the various production processes, and for rinsing out dye residues from machinery. For example, a bleach works requires 50m3 to 100m3 of water per tonne of product, while a dye works uses between 20m3 and 50m3. This volume ends up as effluent, and most of the COD is again organic in nature.
inge watertechnologies is developing a process using UF (see Figure 3) and ultrasound technology for the treatment of dye effluent from the fine chemicals industry. In the Italian textile town of Prato in Tuscany, UF is being tested for filtering out particles and suspended solids from municipal wastewater. Funded with €2.2 million from the EU Commission, the project aims to demonstrate that a combination of UF and ultrasound can replace the more expensive process of RO downstream to a MBR system, by successfully removing dissolved organic components so that the treated water can be re-used. The goal is to establish a method of treating textile effluents that optimises both the cost and the ecological benefits. The study is addressing membrane pore size and geometry, membrane hydrophilicity, determining the best flux and the optimum backflush flow rate, deploying cleaning chemicals and minimising their use.
This test project involves the simultaneous installation of three independently controlled membrane lines to enable the optimum adjustments to be identified as quickly as possible. Peter Berg, chief technology officer at inge explains: “If we succeed in cleaning up textile effluents at a reasonable cost using ultrafiltration and ultrasound, this should lead to a boom in the recycling of industrial effluents. The treatment of organic substances such as dyes is currently tackled by RO. However, this is an expensive method. If UF and ultrasound can provide a better return on investment, then nothing will stand in the way of deploying this new system on a global scale.”
Conclusions
We have seen that fine chemicals comprise a vast array of products from pharmaceuticals and agrochemicals through to specialist chemicals (such as inks, paints and other coatings) and high purity chemicals. We have identified the use of membrane systems using full scale technologies such as UF and MBR systems for the production of process water and effluent treatment, both feeding and arising from fine chemicals production. In processing the chemicals themselves we have found less direct use of membranes with applications highlighted in the production of high purity chemicals for the microelectronics industry. However, advances in membrane technology are continuing to be realised with increased efficiency and synergy with other processes such as ultrasound in dye effluent treatment and EDI for the production of high purity process water. Hence, we can expect the market for membranes within the fine chemicals industry to expand in the medium term.