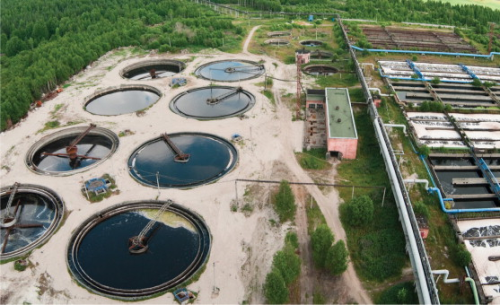
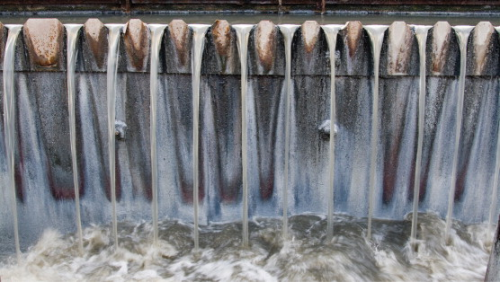
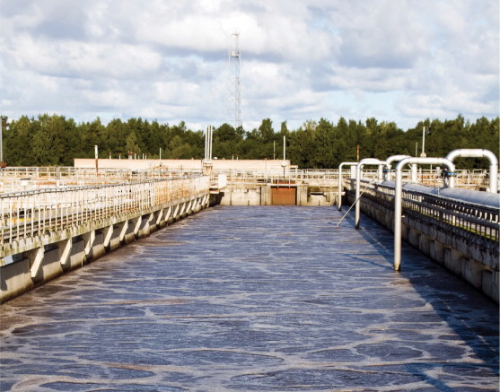
The membrane bioreactor is becoming the process or equipment of choice when it comes to the treatment of a wide variety of waste waters, including those from municipal sources. It has some considerable advantages over conventional activated sludge treatment: excellent quality of treated water, especially in terms of freedom from pathogens; small footprint; replacement of post-digestion settlement and clarification; ease of retrofitting to existing works that are failing or are up to capacity; lower energy demand; and reasonable cost. It also has the potential to be installed, set working and then largely forgotten.
The MBR (including its most common form, the SMBR or submerged membrane bioreactor – not to be confused with the moving bed bioreactor or MBBR) is a fascinating combination of two steps of waste treatment: the bacterial digestion of waste organic matter, and the separation of the treated effluent from residual suspended solids. Its prime advantage is that it can constitute the whole of the secondary phase of the traditional three-phase municipal sewage treatment process, and can eliminate some parts of the tertiary phase.
History
The MBR has developed quite rapidly to become this important. Its acceptance and speed of development are indicated by the fact that it is not mentioned in the 2nd edition of Keith Scott's otherwise comprehensive “Handbook of Industrial Membranes”, published in 1998. It is not even referred to in the 8th edition of Perry's Chemical Engineer's Handbook, published in 2008. Another indicator is that the First International Meeting on MBRs for wastewater treatment was held at Cranfield, UK only 13 years ago, in early 1997.
The idea of combining sludge digestion with a very fine filter was first developed in the mid-1960s, by Dorr Oliver, as a system with flat plate membranes in a sidestream loop. This development was made possible by the appearance on the market of commercial scale MF and UF membranes. In this form, the MBR served niche industrial markets that could afford a rather expensive system producing a low-value product.
The major breakthrough, which allowed the MBR to grow much faster, was the development in Japan in 1989 (as a result of a Japanese government initiative to find better ways of wastewater treatment) of the submerged membrane. The resultant submerged (or immersed) membrane bioreactor used two orders of magnitude less energy than the sidestream version. It was this version that then entered the European market in the mid-1990s.
Since 1990 the numbers of installed membrane bioreactors have grown almost exponentially. There are probably around 3000 plants currently in use, worldwide, although a lot of these are quite small.
The MBR system
The basic membrane bioreactor consists of two processing steps – a bioreactor, in which aerobic bacteria digest organic material in the presence of dissolved oxygen, and a membrane module, through which relatively pure water separates from the suspension of organic matter and bacteria. These two units may be set up to run in succession (i.e. the liquid flows first through the bioreactor, where it is held for as long as necessary for the reaction to be completed, and then through a loop containing the membrane separation stage), with a recycle of some of the separated sludge to the bioreactor. This is often called sidestream (or external) operation.
Alternatively, the membranes are suspended in the slurry in the bioreactor, which is appropriately partitioned to achieve the correct air flows, with the surplus sludge withdrawn from the base of the bioreactor at a rate to give the required sludge retention time (and independent of the clean water offtake rate). This is then termed a submerged (or immersed) MBR.
The membrane bioreactor takes the place of the whole secondary stage of conventional activated sludge processing – and does it better, and in a much smaller space. The excess solids created by the oxidation process can then be easily removed for subsequent treatment. It is a continuous process, and one that is quite easily controlled. It is rapidly becoming the best available technology (BAT) for wastewater treatment.
The sludge settlement stage of the conventional secondary process is a fairly slow one, so the removal of the clear liquid from the slurry is a better option, and results in a cleaner liquid, because of the membrane filtration, at least to microfiltration standards, and more commonly to those of ultrafiltration.
A major advantage of the MBR system is that it can operate at a much higher solids concentration in the bioreactor than that of a conventional activated sludge plant. The MBR plant can work effectively at MLSS (mixed liquor suspended solids) concentrations typically in the range 8000 to 12,000 mg/l (or 0.8 to 1.2%), and has been demonstrated successfully at up to 3%, whereas conventional activated sludge plants work at about 2000 to 3000 mg/l, because of the limitations on settling.
This high sludge concentration capability enables an MBR system to deal effectively with strong industrial wastes, especially in places where water is short, and factories are seeking to close their water cycles. It also results in a much smaller digestion tank, and so a much smaller footprint for the whole plant.
The cost of an MBR plant for secondary processing is falling and approaching that for a conventional plant. As the numbers of MBR plants increase, and as membrane costs continue to fall, the life cycle cost differential will soon disappear, and the process advantages should lead to rapid uptake of the MBR system even by the conservative wastewater treatment industry. The smaller footprint of an MBR plant will make it much more attractive for construction in developed urban areas.
The longer sludge retention times in the MBR permit the destruction of molecules difficult to biodegrade, such as detergents. With proper system design nitrogen and phosphorus contents can also be significantly reduced.
Water quality
As has already been mentioned, the quality of water produced as effluent by an MBR is excellent, and usually well under the local consent limits. Figures of < 2 mg/l for BOD and < 0.5 mg/l for total suspended solids are readily achievable, along with ammoniacal nitrogen of < 0.5 mg/l and turbidity of < 0.2 NTU. Particularly relevant are the capabilities of UF membranes (the most common form used) for removing pathogens such as protozoa, bacteria and most viruses.
Membrane format
Two main formats are used for the membrane material. One, developed by Kubota, uses flat rectangular sheets of membrane, welded in pairs around a frame for each pair, hanging vertically and parallel to other such cartridges on either side of it. Between the membrane sheets and the support frame are spacer sheets that allow the permeate to run from the inside of the membrane to a withdrawal nozzle at the top of the cartridge. Liquid flow is from the outside in to the centre of the cartridge.
The other, developed by Zenon, uses bundles of hollow fibres to form the membrane module. These also hang vertically, with liquid flow from the outside of the fibres in to their centres. The bottom end of the fibre is sealed shut, and the top end together with all of the other fibres is sealed through an end cap into a permeate offtake chamber. The hollow fibre, with this flow direction, is perfectly capable of resisting the relatively low transmembrane pressures entailed in MBR operation, but several manufacturers advertise their use of reinforced fibres (mainly for resistance to cleaning operations).
Either of these formats can be used for submerged or sidestream operation. A third, employing capillary tubes of membrane, is mostly used for sidestream separation, with liquid flow from inside the tubes outwards into a permeate collection chamber. These membranes operate in through-flow mode, with separated sludge collecting inside the tubes, and having to be backwashed out at regular intervals, while the flat sheet and hollow fibre membranes operate in an approximation to cross-flow, with the solids continually flushed away from the membrane surface by an air-scour operation.
A fourth format has the membranes in the shape of circular discs mounted on a horizontal shaft. The whole array is submerged in the activated sludge suspension, and the discs are rotated. Permeate flow is from the outside of the double-sheet discs and in to the central shaft, which is hollow to provide permeate offtake flow.
Another recent format is the coarse tubular membrane, with tube diameters of 8 to 12 mm, while Huber has something totally different in the form of flat sheets, mounted on a rotating hexagonal or octagonal frame. Whichever the membrane format, air scouring is used to remove solids from the vicinity of the membrane surface. This may be the same as the air flow used to activate the solid suspension, or (and especially in sidestream arrangements) the two gas injection systems are quite separate.
Driving force
Most membrane filtration processes require quite a high transmembrane pressure in order to produce an acceptable permeate flow rate. By contrast, the membrane bioreactor operates with a low differential, of about 0.5 bar. This can be provided by a vacuum pump, sucking on the permeate discharge line, through a receiver, or by the hydrostatic head of a deep bioreactor tank, or by a low level of pressurisation of this tank.
The MBR mainly operates by ultrafiltration, but some microfiltration membranes are now used, where the degree of separation is not required to be so high.
The MBR business
The membrane bioreactor business is probably worth around $400 million, and has just one end-use customer: the wastewater treatment business (as well, of course, as all industrial companies producing a liquid waste). This is a huge, worldwide activity, with very large companies (as well as municipalities) taking an increasing part. On the whole, the operators of treatment works tend not to be the suppliers of the equipment used in them. Rather this is done at two further levels: engineers and contractors engaged to build the works and specify the contained equipment, and then the specialist manufacturers of the equipment as appropriate.
The MBR business can thus be found among the wastewater equipment suppliers, either the specialist builders of waste treatment equipment, and especially of secondary processes, who have acquired membrane technology (possibly by buying a membrane specialist company), or the membrane system manufacturers, who have developed an expertise in wastewater treatment. Because the MBR lends itself to a packaged product, then package builders will also be part of the business.
The earliest suppliers were Zenon in Canada and Kubota in Japan, closely followed by Wehrle Werk in Germany. There are now at least 70 acknowledged manufacturers of MBR systems world-wide, of which the largest are:
Asahi Kasei (Microza)
Siemens (USFilter)
GE (Zenon/Ionics)
Suez Environnement (Ondeo)
G L & V (Eimco/Copa)
ITT Aquious (PCI Membrane)
Veolia (Envig)
Wehrle Werk
It is of interest to note that this list contains four of the large global companies that have vowed to make an impression in the water business: GE, Siemens, Suez and Veolia. Among the 70 companies, 14 (20%) are from Germany, 11 from the USA, and 8 from the UK.
The number of MBR installations in the world varies very widely according to source, which probably means with or without the smallest plants. Bearing in mind the inclusion among the suppliers of makers of MBRs for a few PE (population equivalents, where 1 PE not, vert, similar 150 l/d), while among the largest plants under construction is the Brightwater (USA) plant with a capacity of 495,000 m3/day, so that a number quotation would be unfair on the makers of the very large plants. Nevertheless, it is probably true that Zenon, Kubota and Mitsubishi Rayon each have several hundred plants installed (the latter two mostly in Japan).
Developments
In the long history of wastewater treatment (over 110 years), the membrane bioreactor is quite a recent invention, and so, not surprisingly, is still in a period of intense development. Key areas of system investigation include:
• aeration system design, to ensure that energy consumption is minimised, and mixing and scouring maximised;
• the use of enriched air or pure oxygen for aeration (as promoted by BOC Linde);
• the possible use of non-membrane MF media (as with ITRI's NWM MBR);
• the design and manufacture of MBR membranes that can truly resist fouling and so enable a realistic ‘fit and forget’ situation;
• development of an MBR capable of continuous use on ocean-going vessels;
• use of MBR ‘in reverse’ to add gases to the bioreactor, or nutrients in a controlled fashion;
• extraction of specific pollutants from bioreactor zone; and
• development of an MBR for use in greywater recycling.
Perhaps the most exciting development in the MBR system would be its conversion from an aerobic process to anaerobic operation, thus producing more energy (in the form of methane) than the plant consumes. Anaerobic designs have been around since the earliest days of the MBR, such as the anaerobic digestion UF system (ADUF) developed in South Africa, and available from Veolia Envig.
These suggested developments have all assumed that the MBR is to be used to treat wastewater, but there is growing interest in combining an MBR with a reverse osmosis system, in order to produce drinking water.
Finally, there are bioreactors in other industries, especially pharmaceuticals, and, even, brewing, which could be developed into efficient MBR systems, for example the continuous removal of ethanol from a ‘brewing zone’ to avoid poisoning the yeasts.