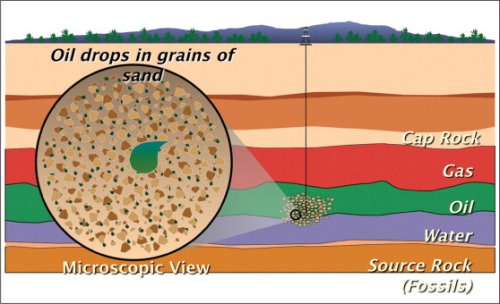
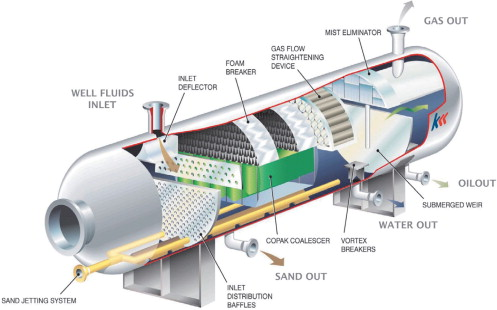
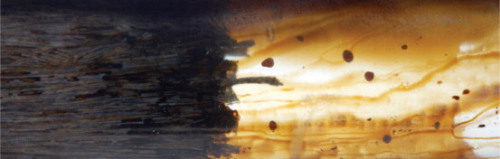
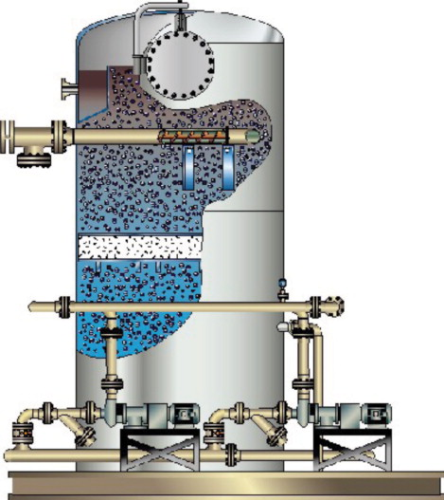
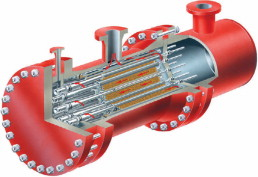
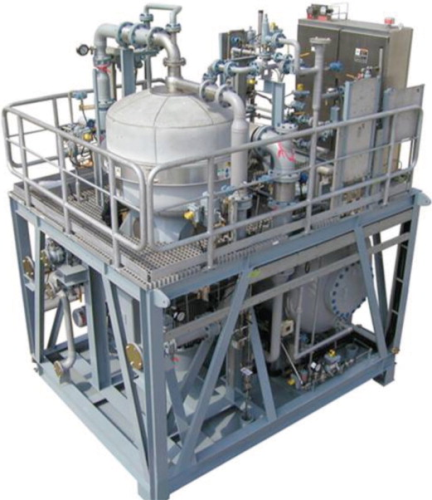
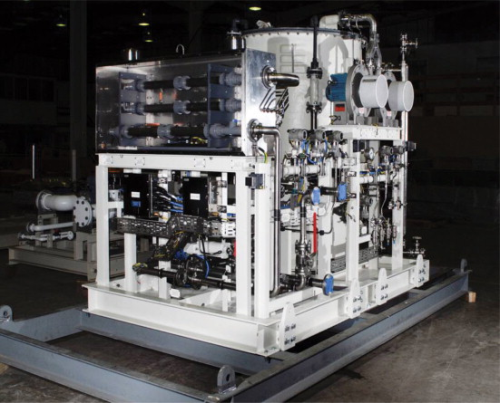
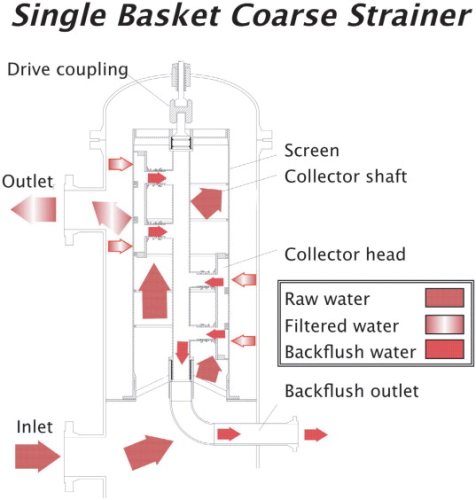
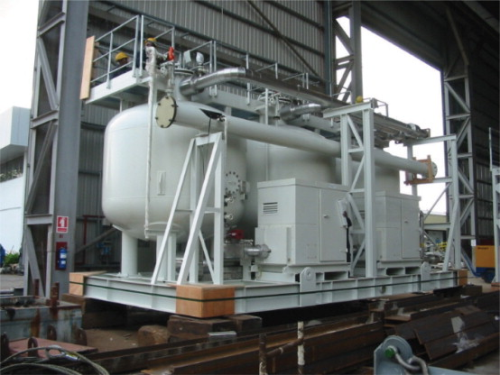
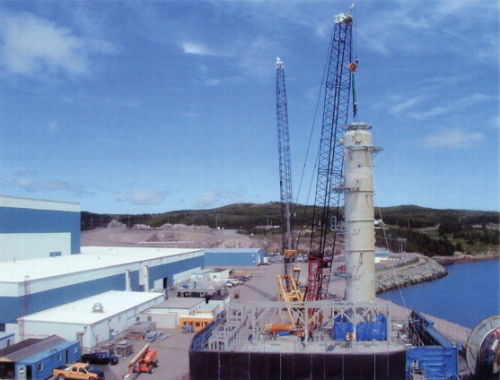
A pdf version of this article is available on the right-hand side under 'Downloads'
Talking with people from outside the oil and gas industry reveals a common conception that reservoirs consist of vast subterranean lakes of the hydrocarbons. The reality is very different as the hydrocarbons are contained in porous layers of rock overlaid with an impermeable rock or shale.
The two major oil-bearing rock types are the sedimentary rocks sandstone and limestone. These rocks are porous with pore sizes ranging from the sub-micron to tens of microns in diameter. Some, but not all of these pores are connected, allowing fluids to pass through the rocks. Figure 1 shows that in addition to the oil, both gas and water are also found in a reservoir.
Typically the water underlies the oil and is often referred to as either ‘connate' or ‘formation' water. These waters differ in their origins. Connate water is the water trapped in the rock during its formation and over its life its composition can change. Formation water is water which has been formed in situ and becomes trapped with the hydrocarbons below the impermeable cap rock. When an oil well is brought into production the oil, gas and water are co-produced.
Oil/water separation
When reservoir fluids (gas/oil/water) are brought to the surface for separation and treatment the pressure is reduced and this sometimes results in the formation of insoluble scales. In simple terms the reduction in pressure allows soluble bicarbonates to be converted to the carbonate ion with the release of CO2 gas:
2HCO3− → CO32− + CO2↑ + H2O
The carbonate ion combines with any calcium ions, for example, to form insoluble carbonate scales. Not only can this result in reduced flowrates (loss of revenue) but this can have an adverse effect on system integrity and needs to be addressed. Inhibition of scale formation can be achieved by dosing a scale inhibitor chemical to the reservoir fluids while these are still at high pressure.
The first stage in the separation of the oil from the other constituents is most often a horizontal three-phase separator (Figure 2), sized so that the residence times of the oil and the water are maximised. Solids are sometimes produced along with the oil, gas and water, so their removal and disposal also need to be considered. All separation of the gas/oil/water/solids in these units is governed by Stokes Law.
The water leaving the separation train is by no means ‘clean'. It will contain some residual oil usually in the form of small droplets dispersed in the water and also possibly some solids. The water will also contain small amounts of dissolved hydrocarbons and gases such as (corrosive) carbon dioxide and the lighter hydrocarbons, as well as any water-soluble chemicals used to optimise the production of the hydrocarbons. So why then does this water need to be treated, as it serves no purpose and has no value at all?
Firstly, the water is most often discharged to the environment – to the sea from offshore oil and gas production platforms, to local river courses from onshore production systems or to estuarine or near coastal waters from oil terminals or oil refineries. With increasing pressure on protecting the environment the presence of potential pollutants in these waters has to be addressed. Secondly, the water does contain some hydrocarbons which could be recovered and returned to the main production system.
Deoiling produced waters
In the early days of treating these waters the systems used tended to be based on mineral treatment technologies, which removed the larger oil droplets but not the smaller ones. Advances in the technology for removing dispersed oil droplets from produced water have centred on the following methods;
a) increasing the overall droplet size (coalescence) (Figure 3);
b) systems which change the specific gravity of the oil droplet by attaching to it a bubble of gas (Figure 4);
c) techniques that apply increased gravitational forces to the separation process, for example, hydrocyclones (Figure 5) and centrifuges (Figure 6).
These advances in reducing the levels of residual hydrocarbons in the produced waters mean that, most often dispersed oil-in-water (OIW) levels as low as 40 mg/l can be achieved. In the early days of North Sea offshore operations this was sufficient to meet the standard for discharges set by the relevant UK regulatory body. More recently the direction of the legislation has changed with offshore operators now required to reduce the annual tonnage of hydrocarbons discharged overboard.
This mass of oil is to be 15% lower than the tonnage discharged by the individual assets in 2001, as the base line. For any hydrocarbon discharged in excess of this the operator has to pay a fine, currently £108 for every kilo discharged in excess of the permitted amount. No allowance is included in these figures for any new fields brought on line or the fact that the volumes of water produced over the life of a field usually increase.
The averaged maximum OIW value is now 30mg/l in the produced waters discharged overboard. Thus in order to meet the new requirements some operators are having to treat their produced water to much higher standards than before and several operators have internally introduced a zero produced water discharge target for both existing and new assets.
Discharges from onshore operations are governed by the relevant environmental protection agency and may include maximum levels for heavy metals and certain dissolved hydrocarbons in their discharge standards.
Other waters
From Figure 1 it can be seen that as the oil is produced two other mechanisms occur as the pressure reduces. These are expansion of the gas cap and an increase in the level of the oil/water interface. The first mechanism is undesirable as the reduced pressure can also allow gas dissolved in the oil to come out of solution. As the gas is more mobile than the oil it will flow preferentially toward the production wells.
This is highly undesirable as it means that oil which could have been produced is bypassed and left in the reservoir. An increase in the level of the oil/water interface is also undesirable as this will lead to increasing levels of water being produced along with the oil. This poses two distinct problems, firstly a reduction in the oil revenue and secondly, a larger volume of water to treat before it can be discharged.
How can these mechanisms be prevented, or at least delayed significantly until the revenue from the field has been maximised? As far back as the early American oilfields the beneficial effects of allowing water to flow into oilfields were discovered by accident[1]. In those days the water often found its way by accident into the oil-bearing strata and acted to flush the oil towards the production wells. Since then knowledge has increased significantly and nowadays very few oilfields are brought into operation without some form of water injection.
The injected water can serve two purposes; firstly it maintains the pressure of the reservoir at a level at which gas cannot break out of solution and secondly it forms an immiscible flood front pushing the oil towards the production wells. Whichever mechanism holds for the reservoir under consideration, there will be a significant overall increase in the amount of oil recovered. The magazine World Oil estimated that based on worldwide average figures a successful water injection operation can improve overall recovery of hydrocarbons by an average of 40%.
The waters most often available for injection into hydrocarbon formations include:
• Seawater (if the asset is offshore or near the coast with a few exceptions);
• Produced Waters (see above);
• Aquifer waters (if easily accessible);
• River or estuarine waters;
• Domestic and/or industrial waste waters.
A significant exception for seawater is the Saudi Aramco Qurayyah system where initially 7 million barrels of seawater per day (1.1 million m3/day) are treated and then pumped between 350 and 400 km inland for water injection into the Ghawar oilfield. Since its start-up in 1978 the plant has been upgraded to twice its initial capacity (completed in 2009) and now also services the Khurais oilfield.
All of these require some form of treatment before the water can be safely and continuously injected into a hydrocarbon-containing formation.
Injection water treatment
By far the most commonly used waters for injection purposes is seawater and the current discussion of injection water treatment will focus on this.
Seawater contains suspended solids, bacteria and dissolved oxygen, all of which can have an adverse effect on either the reservoir's ability to sustain long term injection of water or on the long-term life of the materials used to handle the injection water. These materials include pipelines, injection well goods and any metals used sub-surface to distribute the water to the reservoir. As the seawater may also have other uses, such as cooling, it must, as a minimum requirement, initially meet the quality needed for cooling. This means removal of bacteria, marine life and treatment to remove the larger suspended solids will be needed.
Bacteria are dealt with by dosing a broad spectrum bactericide, usually chlorine as sodium hypochlorite, to the pumps feeding the water to the asset. This is normally generated by electrolysis of the seawater (see Figure 7). Following this the larger suspended solids such as the hard–shelled marine organisms and plankton are removed by means of coarse filtration (Figure 8). These are designed to remove a range of solids depending on how the water is to be used. Solids removal typically can vary between 80 μm and 6.4 mm. After cooling duty, the water may require further filtration for injection into the hydrocarbon-containing formation.
On this topic there are currently two very different schools of thought; one which advocates filtration to prevent blocking of the reservoir pores, the second believing that the cold seawater entering the hot rock will generate fractures in the rock thus allowing the water (and solids) to flow freely[2]. Should secondary filtration be required, then a bank of high-rate dual media downflow filters (Figure 9) is most often used. Having dealt with both solids and most, but not all of the bacteria, the next area where treatment is required is the removal of the dissolved oxygen.
Since carbon steel is the preferred material for handling the high pressures required to inject the water into the formation and is prone to corrosion by the oxygen in the water, the dissolved oxygen must be removed. The commonly-used technology for removing this oxygen from the seawater is by means of a vacuum deaeration system, comprising a vertical vessel containing multiple vacuum stages (see Figure 10) which will reduce the level of oxygen in the seawater from not, vert, similar8 mg/l to <50 μg/l. The residual dissolved oxygen is removed by the chemical reaction of a sulphite-based scavenger chemical.
SO32− + O2 → SO42−
The various stages of vacuum are produced by means of liquid ring vacuum pumps, typically operating at the first stage's level of vacuum, with air/gas ejectors used to provide the lower vacuums. Seal/cooling water for the pumps is usually cold coarse filtered seawater. The conditioned seawater then has its pressure boosted before being distributed to the water injection wells. With the change in conditions from aerobic to anaerobic downstream of the deaerator, this can provide conditions where some anaerobic micro-organisms, such as the sulphate reducing bacteria, can proliferate with potentially disastrous results to the integrity of any carbon steel systems[3].
This type of microbiologically influenced corrosion (MIC) is usually mitigated by the intermittent dosing of organic, non-oxidising biocides. Unlike chlorine, these products are not corrosive. Chemical biocides such as aldehydes (glutaraldehyde and formaldehyde), quaternary ammonium compounds and some specific types of quaternary phosphonium compounds are dosed singly or as blended products. Organic biocides are expensive and are usually dosed weekly for a period of 1-2 hours at dose concentrations up to 1,000 mg/l.
The actual dosing frequency, duration and dose rate are adjusted based on the results of monitoring the water quality downstream of the biocide dosing point for both planktonic and sessile bacterial species. Finally the water being injected may form insoluble scales either when its temperature and pressure are raised to that of the hydrocarbon-containing reservoir or when the seawater and the formation water with which it mixes as it passes through the reservoir are chemically incompatible. Depending on the magnitude of the problem – and there are several ‘expert' software programmes to predict this accurately – the addition of a scale-inhibiting chemical to the injection water may be sufficient.
For example, where the formation water is high in terms of the dissolved higher alkaline earths (especially barium and strontium) and the water being injected is seawater with a typical sulphate ion concentration of the order of 2,700 mg/l, then there can be a severe risk of the formation of insoluble barium and strontium sulphates. The amount of these scales forming can often be too high for an effective chemical scale inhibitor treatment. One technique commonly used is to pass the seawater through a bank of nano-filters, which effectively reduce the levels of divalent ions, such as sulphate. This allows the treated water to be safely injected into reservoirs with high barium/strontium levels, with no adverse long-term effects on injectivity or production.
Obviously injection water treatment is a major process. However, a most important aspect of any system is to make sure at the design stage that the operations team has an input. The operations team will have to make the system perform to specification and rarely is the designer involved in commissioning/operating of the system.
Conclusions
There are many reasons why water treatment is a vital and integral part of both oil and gas production operations. Inadequate design of the water treatment system, or forgetting to optimise the system's operation, could lead to a shutdown of the hydrocarbon production system. Such a shutdown might have to last until the problem has been resolved, with associated significant loss of revenue.
References
1 “The Reservoir Engineering Aspects of Waterflooding”, FF Craig Jr. SPE Monograph Volume 3.
2 “Thermally Induced Fracturing of Ula Water Injectors”, Svendson, A.P., Wright, M.S, Clifford, P.J., Berry, P.J, BP, SPE Paper 20898-PA, 1991.
3 “Safety Aspects of Water Injection Facilities”, Robinson K., Oil Plus Limited, 3rd International Conference on Water Management Offshore, Aberdeen, UK, Oct 1993.
Uncited reference
Glossary of oil and gas field terminology – http://www.glossary.oilfield.slb.com/ (courtesy of Schlumberger).