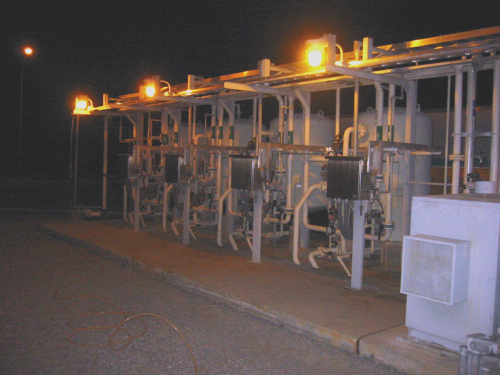
Recovery of wastewater for industrial use presents many challenges for the design and operation of water treatment equipment. Wastewater has high levels of dissolved solids, and higher metals content than in natural water sources and potentially high levels of organic matter, both natural and manmade. At a power plant in Mexico, the wastewater treatment system was fed with secondary water used in the production of boiler water makeup water. This is a gas-fired combined cycle plant with a generating capacity of 600 MW and has been in operation since 2002. The plant obtains its water from municipal primary treatment lagoons/settling ponds. The main source of water entering the lagoons is municipal sewage.
Secondary treatment of the water is provided at the power plant itself. The sewage treatment plant uses a biological treatment process to first oxidise organic matter and NH3 in an aerobic step and then remove nitrates, formed by NH3 oxidation, by bacterial action under anaerobic conditions. This eliminates biological contaminants and reduces other contaminants in the water. The water is then treated with lime, which raises the pH of the water, which causes the precipitation of dissolved minerals such as calcium and magnesium and reduces the overall dissolved solids content.
The water treatment plant originally consisted of sand filtration, cartridge filtration, two-pass reverse osmosis (RO) and electrodeionisation (EDI). The plant needed additional capacity and was also experiencing operational difficulties. RO units fouled rapidly and required frequent cleanings and the EDI system was not consistently producing water of sufficient quality. After extensive evaluation, the system supplied by manufacturer of water purification and chemical recovery equipment Eco-Tec consisted of the following elements:
• Pretreatment using a single high efficiency micro media filter
• Upgraded RO
• Compressed short bed IX demineraliser.
Pretreatment
Secondary water sources cannot be used directly as feed to RO systems because they contain high levels of suspended solids, organics, colloids and biological material even after clarification. A tertiary treatment process is required to prevent fouling of the RO membranes. The original system consisted of two trains of two-stage media filters. Performance of this filtration system was poor and showed frequent spikes in the solids content of the filtrate. Cartridge filters following the media filters, were overtaxed, and solids leaked through to the RO having an adverse effect on performance, and increased the need for membrane cleaning.
The two-train system of primary and secondary media filters was been replaced with a single micro media' dual-media filter, which differs from typical dual media designs. While the top media layer consists of coarse anthracite similar to that used in many conventional dual media filters, the lower ‘micro media’ layer is a high density media, significantly finer than the fine media used in most designs which has an effective size of about 0.35 mm.
Since the lower layer removes the residual quantity of suspended solids not retained in the upper layer, it effectively defines the filtration efficiency. While the higher service flow and finer media result in larger initial pressure drops across the filter, the pressure drops are manageable. Due to the higher flow rate, the diameter of the filter vessel required to treat a given flow of water can be reduced. The single micro media vessel is 5.5 ft in diameter and has a design service flow rate of 15 gal/min/ft2.
To maximise the cleaning efficiency of the backwash, the micro media filter operates with a simultaneous air scour/backwash cleaning cycle. Water is drained to the top of the media. Air and water are then passed simultaneously up through the media bed. The water flow expands the media and allows the air to agitate the media much more violently and uniformly than with water alone. The vessel is then refilled with water, the air is turned off and a water backwash flushes dirt from the filter in the usual manner.
Comparing performance
A performance comparison was carried out between the existing sand filter trains and a pilot-scale micro media filter (6 inch diameter column). The feed to the system was the same as that to the sand filters, the feed stream being split after the point of coagulant addition.
Effluent turbidities over a short period and the inconsistent performance of the sand filters was apparent. The initial point of upset for the sand filters coincided roughly with the time when one filter was brought back into service after being backwashed. It is also important to note that the coagulant dosage was not at the point required for optimal performance, and this is most probably the reason for the filtrate turbidities never falling below 0.2 NTU.
Reverse osmosis
The existing RO system was configured into two trains, each including three stages and producing 14.2 m3/h. A sample of permeate from the RO taken just prior to the upgrade indicated a conductivity of 392 μS/cm. At the time of the analysis the RO membranes were approximately three years old. The poor permeate quality was attributed in part to leaky seals between the membrane elements and membrane degradation due to fouling of the membrane.
The upgrade involved replacing the existing membranes with higher surface area membrane elements. The permeate flow rate per train was increased to 28.4 m3/h per train. The original membrane configuration used membrane elements with a 7% lower surface area and a flux rate of 7.5 gal/ft2/day, whereas the upgraded system operates at a flux of 14 gal/ft2/day. Despite the increase in fluxes the membranes have experienced less fouling since commissioning and the upgraded system produced permeate with a conductivity ranging from 30–70 μS/cm.
Compressed short bed ion exchange
The original system utilised EDI stacks, typically used to treat double pass RO permeate. The plant decided to replace the EDI cells and expand capacity using a packed bed ion exhange (IX) system. The technical considerations for this decision were the rapid start-up after shut down provided by the packed bed system, elimination of the second pass RO requirement, and operating/maintaining a single type of polishing system.
Standard onsite mixed bed systems have a long and proven history of successful performance. Relative to newer EDI and packed bed technologies, operation and regeneration can be much more complicated, particularly when resins age. Many of these problems are related to resin separation and uniform re-mixing before and after regeneration.1 Another disadvantage of mixed bed technology is the high regenerant consumption and wastewater production.
Packed resin bed technology reduces these problems by using separate beds of cation and anion resin that are counter-currently regenerated. Separating the resins eliminates the separation and mixing problem. Counter-current regeneration minimises regenerant consumption and produces high quality water by ensuring the highest degree of resin regeneration at the bottom of the column. The cleanest resin is the last to contact the product water ensuring the lowest contaminant levels.
A further refinement to this technology is the compressed short bed system,2 with a short bed height of 3 inch or 6 inch. Other features include a much smaller resin bead and complete elimination of internal freeboard. The smaller bead size greatly improves the kinetics of the exchange process, which allows operation at higher flo w rates and reduces the depth of the active exchange zone. Eliminating freeboard and operating the resin in a slightly compressed state ensures that flow is uniformly distributed and that the resin position is maintained during regeneration in order to obtain full benefit of the counter-current operation. One of the main concerns when using packed bed systems is resin fouling. Since resin is not backwashed during regeneration, any accumulated dirt will not be removed, hence the need for highly efficient pretreatment. With this in mind, treatment of RO permeate by packed bed systems is an ideal application.
When using separate beds of cation and anion resin the limiting factor with regards to product water quality is generally sodium leakage from the cation bed or from residual caustic left on the anion resin after regeneration. This becomes problematic when trying to produce high quality water (<0.1 μmho/cm) and with higher feed TDS (>25 mg/L).
Given the feed composition and product water quality target a cation/anion bed combination is not sufficient even with a compressed short bed system, a third polishing bed of cation resin is added to remove low levels of sodium. This low level allows the use of a bed only three inches deep, operated at an even higher flux with very infrequent regeneration. A single train skid-mounted compressed bed system with cation/anion/polishing cation beds was supplied.
Upgrade successful
Altogether, an upgraded and expanded water treatment plant was supplied to treat a secondary wastewater stream. A high purity product water with a conductivity <= 0.6 μmho/cm was produced and supplied as boiler make-up water. Tertiary treatment is provided by a high efficiency media filter replacing existing sand filters and was found to be more economic than ultrafiltration. This filter typically produced filtrate with a turbidity 0.1 NTU and SDI values of <3. Primary demineralisation is provided by a single pass RO system using low fouling composite membranes. Permeate quality ranges from 30 – 75 μmho/cm when treating feed water with a TDS of 700 – 1,000 mg/L. Polishing is provided by a compressed short bed IX system, which replaced existing EDI cells. The new system is able to operate on a feed with a much higher TDS eliminating the need for second pass RO. The problem of slow EDI start-up after shut down was also addressed. All performance objectives were achieved and the system has now been in service for over a year without any problems.
References
1 Smith, J.H, Renouf, P.W. and Crossen, M. – 50 Years in Separate Beds, Technical Paper presented at the International Water Conference 1984.
2 Sheedy, M. and Kutzora, P. – The Use of Short Bed Ion Exchange Technology for the Product of High Purity Water at WE Energies PPPP, Technical Paper presented at the International Water Conference 2004.