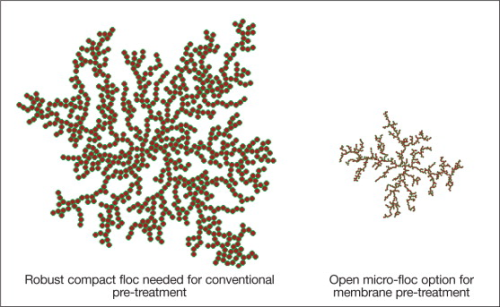
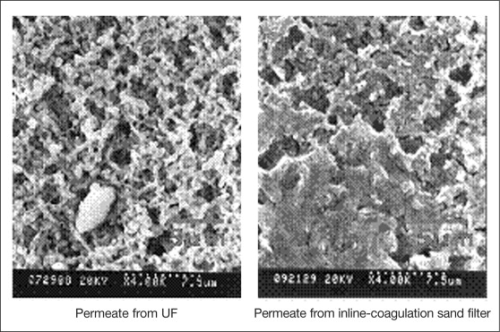
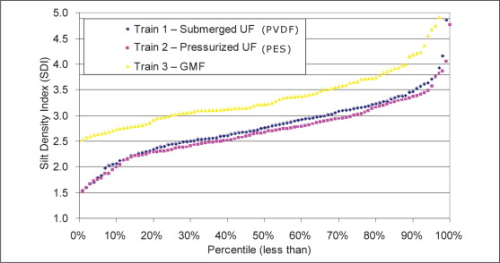
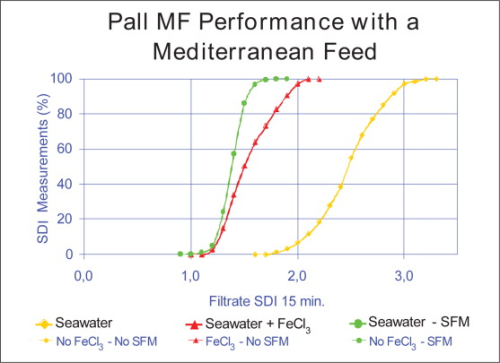
A pdf version of this article, as well as the second article in the series, are available on the right-hand side under 'Downloads'.
Background
Selection of pre-treatment technology for desalination in seawater reverse osmosis (SWRO) applications tends to polarise opinion. Mistakes in design or operation of the pre-treatment system can have serious consequences for the RO plant, both in terms of meeting quality and output targets, and in cost, longevity, and environmental impact. Conventional technology represents the status quo and is known and trusted technology, albeit with acknowledged shortcomings. Meanwhile, membrane filtration adopts the role of the challenger, with enthusiastic proponents due to the advantage it offers as a barrier technology.
Despite a rapidly growing uptake in new projects, there are two issues that limit the wider adoption of membrane pre-treatment. Firstly, it is considered expensive in terms of capital cost, though it does provide operational cost savings. Secondly there are misgivings about whether membrane filtration alone provides sufficient pre-treatment, since preparing a feed suitable for RO requires more than the removal of fine particulates.
When consulting the literature the decision on pre-treatment technology is not clear cut, because papers can be found to support both options. For example, Montgomery Watson Harza showed that conventional pre-treatment at Point Lisas performed well on a Caribbean feed, and was selected for the main plant [1]. However, ultrafiltration (UF) was also found during pilot trials at this site to offer potential advantages of lower chemical use [2], but was discounted for the original 2002 installation due to a lack of UF pre-treatment references at this time.
More recently, the US Bureau of Reclamation showed that conventional pre-treatment of the tidal Anclote River was not sufficiently robust for a highly variable feed influenced by surface run off. The improved reference position for membrane pre-treatment by the time of this study in 2008 prompted the selection of microfiltration (MF) for project development [3]. Elsewhere, the Affordable Desalination Consortium (ADC) in California experienced problems with conventional pre-treatment during poor feed quality episodes, but found membrane pre-treatment to be more reliable and consistent [4].
However, several projects have switched their pre-treatment choice during the evaluation phase. For example, Thames Water initially selected conventional pre-treatment for its Beckton plant in the tidal Thames estuary in 2004, but took advantage of a 2½ year project delay to switch the decision to UF pre-treatment since the reference positioned had improved [5]. Conversely, Poseidon initially selected membrane pre-treatment at Carlsbad in California after extensive piloting [6], but now appears to prefer conventional pre-treatment [7].
This article is the first in a series which will examine the issues involved in the selection of pre-treatment technology for SWRO, and consider the case for conventional and membrane pre-treatment options. The first article will examine the issue of the treated water quality of the RO feed. Subsequent articles will cover Integrity and Disinfection, Cost and Sustainability, and Markets and Experience. The articles will focus on the comparison between pre-treatment technologies.
Pre-treatment objectives
The RO spiral wound element is unfortunately highly susceptible to fouling, and hence feeds with a high fouling potential such as open intake seawater require extensive pre-treatment [8]. Particles clog the feed spacer, low molecular weight organics adsorb onto the membrane surface, inorganics precipitate in the feed channel, and bacterial organisms cause bio-fouling. Fouling by inorganics can be controlled by stabilising the feed with pH adjustment and other dosing chemicals. Controlling the other causes of fouling requires pre-treatment.
Filtration is used to control fouling by particles. Clearly, the finer the filter rating, the more effective the particle removal will be. Control of organic fouling is more difficult, since the species of interest may be very low in molecular weight. The classic approach to this problem is to remove organics by coagulation, normally with iron salts. Bio-fouling control requires the removal or destruction of bacteria, and the removal of its food source. It therefore requires a combination of coagulation and disinfection or inactivation. Bio-fouling will be discussed in more detail in the next article.
Marine organisms are an important category of particle foulant, and include animal species such as mussels etc, and vegetation such as algae or red tide. To some degree, these organisms can be treated as inanimate particles and removed by filtration, especially if the feed is pre-chlorinated. However, if cells are ruptured by the pre-treatment process, organic chemicals may be released which can have a profound effect on the pre-treatment system and the RO. In addition, pre-chlorination can have a severely detrimental effect by creating more readily digestible forms of carbon known as Assimilable Organic Carbons (AOCs) and potential carcinogens known as Disinfection By-Products (DBPs). On occasion, population explosions may occur such as algal blooms or red tide events giving rise to an occasional overwhelming challenge. In consequence, a clarification and settlement stage may be specified in order to ensure sufficient robustness in the pre-treatment system to cope with poor feed quality episodes.
Countering the effect of fouling
An RO membrane allows water to permeate under a pressure differential while retaining salt. However, a low level of salt passage also occurs even with a new membrane. Salt passage is enhanced by minor defects from a low level of mechanical damage during normal operation. This may occur due to the impingement of particles on the surface or by the movement of the feed spacer across the surface caused by pressure surges in the system. This effect increases slightly with time due to natural hydrolysis of the polymer chains, thereby limiting the selectivity of the membrane.
Replacing a proportion of the RO membrane elements counters the degradation effect and enables the plant to achieve a stable permeate salinity. This procedure is known as RO element replacement, and might occur at a level of 8-20% of the element inventory each year for an established plant to ensure consistent output quality.
Salt passage, or the degradation of selectivity, is affected by the quality of feed water being treated. It is clearly desirable to remove particles and colloids from an RO feed since if present, they may cause mechanical damage to the membrane surface, or eventually clog the feed channels. In addition, the adsorption of low molecular weight organics onto the membrane surface tends to increase the rate of salt passage. Furthermore, the RO membrane is highly sensitive to chemical degradation both from oxidising chemicals, such as chlorine used for disinfection or bio-fouling control, or RO cleaning chemicals. The active layer of the RO membrane is attacked by oxidising or cleaning chemicals, and the resulting damage causes an increase in salt passage, which gives a permanent loss in selectivity.
Thus an RO plant without an element replacement programme would suffer from a slow steady decline in selectivity, punctuated by step changes due to chemical degradation. A low element replacement rate would counter the combined effect of the intrinsic selectivity loss and an occasional chemical clean; a high replacement rate would be required to compensate for the additional effect of more frequent chemical cleans and the occasional risk of exposure to oxidants. In addition to the cost of chemicals, cleaning incurs downtime and creates waste for disposal, thus improvement in RO feedwater quality is desirable on several fronts.
The role of coagulation
Coagulants are widely used in seawater pre-treatment. In conventional treatment, coagulants are critical for rendering fine particles filterable. In addition, dissolved organics become associated with the growing floc particles and when removed, reduce adsorptive fouling effects.
Membrane pre-treatment is less reliant on coagulation for particle removal due to its fine pore size rating. However, coagulants have the desirable effect of improving dissolved organic removal, and reducing the fouling rate on the pre-treatment membrane itself, which allows use of higher flux rates and lower cleaning frequencies [9]. Since the membrane is not as dependent as a multi media filter on the formation of large robust floc particles for good removal efficiencies, low dose concentrations and short contact times can be employed [10], as illustrated in Figure 1.
A key benefit of coagulation is association of the floc particle with algal cells, or other marine organisms which makes them more filterable, and less prone to stick to the surface of the pre-treatment membrane or media. Low density organisms are efficiently removed in a flotation process [11], whereas higher density particles, particularly inorganics, are best removed in a clarifier since the floc particle has more of a tendency to settle rather than float.
Coagulants may be added either through a clarification or flotation stage for both conventional and membrane pre-treatment. Membrane pre-treatment offers the additional option of in-line dosing, though depending on feed characteristics, the effectiveness may vary [12].
Indicators of treated water quality
The presence of particles in an RO feed can be indicated directly by measuring total suspended solids (TSS) or particle counting, or indirectly by turbidity. Dissolved organics can be indicated by measuring total organic carbon (TOC), or by specific analysis for particular components. Although these analytical tools are used to characterise RO feed quality, the industry still relies heavily on an analytical measure that has been developed through custom and practice that is known as the Silt Density Index (SDI) measurement. A development of this concept is also occasionally used, namely the Modified Fouling Index (MFI).
SDI and MFI measurements are used for evaluating feed water quality to RO, both in terms of particulates and dissolved components. Normally, indices are measured off-line, in which case the equipment required is simple and straightforward, though there are on-line versions. The main benefit of using these indices is that they are easy and inexpensive to use, and provide a measurement of reasonable accuracy. However, the commonly used SDI has significant limitations, and it is important that these are understood.
SDI uses a 0.45 μm membrane disc to evaluate water quality. Pressure is applied to the sample upstream of the disc and the rate of water flow through the disc is measured. By monitoring the decline in water passage through the disc with time, a plugging factor is evaluated from which the SDI is calculated. The index is affected by pore plugging and cake filtration as solids build up. MFI uses a similar principle, but provides a measure of fouling by cake filtration only, due to the method used for analysing the data [13]. Fouling in RO is not affected by pore plugging, but is mainly determined by cake filtration, especially if a high solids condition develops in the feed channel, and therefore MFI is a more suitable technique to elucidate fouling propensity. Of course RO fouling is also affected by other mechanisms such as adsorption, concentration polarisation, scaling, and bio-fouling, but these effects are likely to be more influenced by dissolved components and less so by solids, and are less relevant to SDI or MFI measurement.
Care needs to be used in measuring the indices, SDI in particular. SDI is affected by temperature, the presence of air bubbles, and the nature of the material of the SDI test disc, including factors such as permeability hydrophilicity, and thickness of the test disc [13]. In addition, the operator has a significant influence on the test result, and so caution has to be exercised in comparing values.
Measuring SDI after UF/MF pre-treatment is fraught with potential error since the test is designed to measure the residual fouling of a 0.45 μm disc from particles. Although conventional pre-treatment is likely to allow some particle passage to allow the index measurement to work, membrane pre-treatment is a barrier to particles that would form a cake layer on a 0.45 μm disc. SDI post UF/MF pre-treatment is determined by fouling mechanisms such as adsorption or in depth pore plugging from small particles, perhaps combined with the effects of UF/MF integrity breaches or sample contamination. It is therefore not a well designed technique for the evaluation of the effectiveness of membrane pre-treatment.
Performance
Conventional pre-treatment if well designed and operated can achieve low particle counts of for example 1 — 10 counts/ml > 2 μm. However, it is quite common for conventional treatment to provide filtrate with particle concentrations an order of magnitude greater than this range, and it is well established that filtrate quality declines badly at the beginning and towards the end of the filtration cycle, and also if there are flow surges or pressure shock. In contrast, membrane pre-treatment is an essentially complete barrier to particles. Figure 2 illustrates the difference in SDI discs between conventional and UF pre-treatment, demonstrating the stark contrast in fine particulate contamination.
The benefit of the lower particulate level in the UF filtrate is that the RO cleaning frequency will be reduced, and more aggressive RO system design conditions can be adopted [8]. Figure 3 shows how the performance benefit manifests itself in terms of SDI measurements [14]. Two different UF technologies were piloted alongside a comprehensive conventional pre-treatment system, both achieving just over a 0.5 SDI unit performance advantage.
MF normally achieves similar SDI results to UF. Figure 4 shows results from an MF pilot [15], illustrating the performance with an uncoagulated seawater feed, a sweater feed with ferric coagulation, and finally with ferric coagulation combined with a Specific Flux Maintenance (SFM) programme. The figure illustrates the beneficial effect of coagulation and process optimisation in improving filtrate quality, presumably due to enhanced removal of dissolved organics.
Conclusions
• Conventional pre-treatment is often used for seawater RO systems, and can operate well, though with acknowledged shortcomings.
• Membrane pre-treatment provides a superior RO feed quality in terms of particulate removal, and is becoming more widely accepted.
• In addition to particulate removal, pre-treatment also requires removal of dissolved organics, which can be achieved by coagulant addition.
• Membrane pre-treatment can operate with smaller and weaker floc particles, which allows lower coagulant doses and shorter contact times, potentially offering the option of in-line dosing.
• RO systems use a membrane replacement strategy to maintain steady performance; since membrane pre-treatment gives an improved pre-treatment quality, lower RO replacement rates and reduced cleaning frequencies can be used, providing significant operational benefits.
References
1 JG Jacangelo and J Grounds, Properly designed conventional pre-treatment provides suitable water quality for RO, Desalination and Water Reuse 13/4 (2003), pp. 16–23.
2 UF for Seawater RO Pre-treatment. Galloway, M, Mahoney, J, Membrane Technology (Elsevier), (Jan 2004) 5-8.
3 Pre-treatment and Design Considerations for Large Scale Seawater Facilities. Reiss, C R, Robert, C, Dietrich, J, Mody, A for US Bureau of Reclamation. Desalination & Water Purification R&D Program Report 137 (Feb 2008) 99.
4 Optimizing Lower Energy Seawater Desalination. Dunsdorf, S, MacHarg, J, Seacord, T F (Affordable Desalination Consortium) Conference Proceedings, IDA Gran Canaria, MP07-150 (2007).
5 An Overview of the 150,000 m3/day Beckton Desalination Plant in London. Moore, B J, Malfeito, J J, Conference Proceedings, IDA Dubai, (2009).
6 UF + SWRO comes of age. Pankratz, T, Water Desalination Report, 43/23 (18 June 2007) 2.
7 Choosing Between Conventional and Membrane Filtration, Voutchkov, N, Desalination (a Filtration & Separation publication), 4/1 (2009) 5-8.
8 Introduction to membranes: Water and Wastewater - RO pre-treatment. Pearce, G K, Filtration and Separation (September 2007) 28-31.
9 KY Choi and BA Dempsey, In-line Coagulation with Low Pressure Membrane Filtration, Water Research 38 (2004), pp. 4271–4281. Article | PDF (379 K) | View Record in Scopus | Cited By in Scopus (39)
10 Surface Seawater Pre-treatment Upstream of RO: Long Term Testing using UF. Bonnelye, V, Brehant, A, Sanz, M A, Perez M, Conference Proceedings, IDA Bahamas, BAH03-062 (2003).
11 A Braghetta, JG Jacangelo, S Chellam and ML Halaling, DAF Pre-treatment: Its Effect on MF Performance, Utne, Journal AWWA 89/10 (1997), pp. 90–101. View Record in Scopus | Cited By in Scopus (27)
12 Determining the Effects of Coagulant Pre-treatment on UF, Sharp, M M, Escobar, I C, Conference Proceedings AWWA Mem Tech, Phoenix, Tues 1-6 (2005).
13 S Boerlage, Understanding SDI and Modified Fouling Indices, Desalination & Water Reuse 18/1 (May/June 2008), pp. 12–21.
14 Extended seawater RO testing in estuarial waters, R Huehmer et al, Conference Proceedings AMTA, Las Vegas (July 2007).
15 D Vial, G Doussau and R Galindo, Comparison of 3 Pilot Studies using MF for Mediterranean Seawater Pre-treatment, Desalination 156 (2003), pp. 43–50. Abstract | PDF (690 K) | View Record in Scopus | Cited By in Scopus (5)
The author has contributed the sections on membrane filtration in The Guidebook to Membrane Desalination Technology and The Guidebook to Membranes in Wastewater Reclamation.