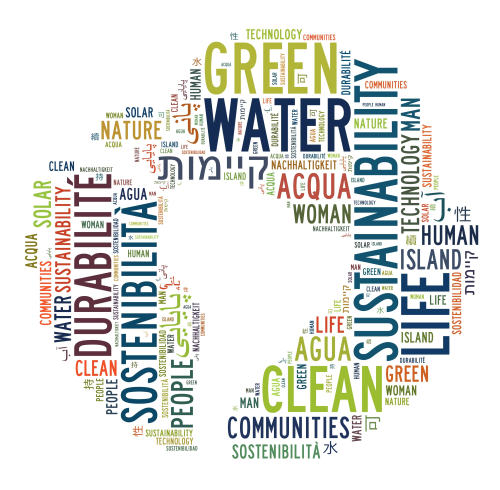
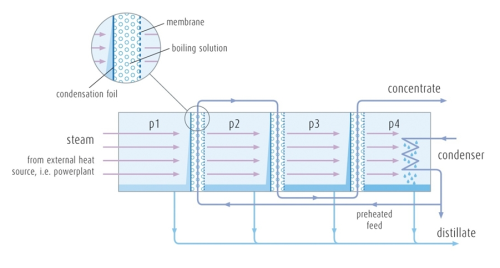
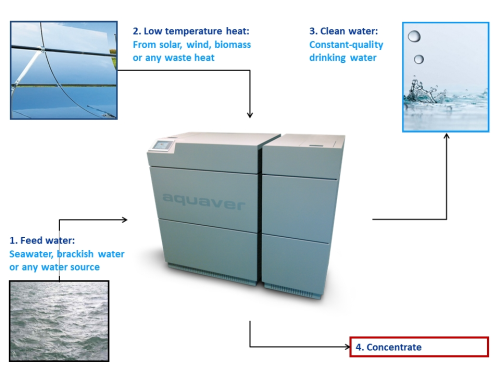
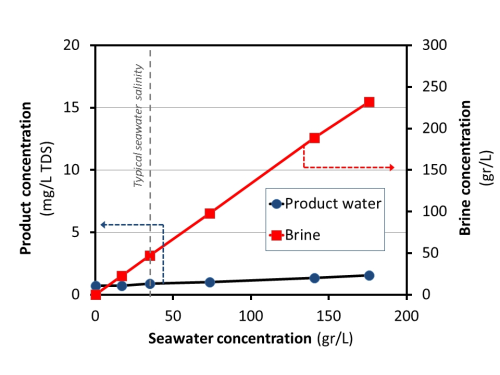
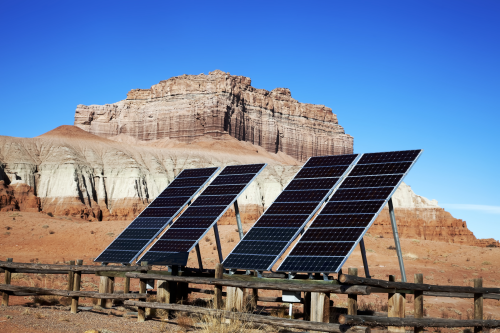
Although 70% of the planet is covered by water, only a very small amount of it is suitable for drinking. An estimated 1.2 billion people globally still do not have access to clean water [1], and nearly 80% of the world’s population is exposed to high levels of threat to water security [2]. A growing population worldwide, increased industrialisation and climate change worsen the situation further. Although there is plenty of water around, most of it is saline or polluted, and needs treating before use. The most common technology for desalination is filtration, a mechanical separation technique in which water is pressurised over a selective membrane. Impurities, such as salts, are retained on the pressurised side of the membrane and clean water is allowed to pass to the other side as product. The high pressures involved to overcome the osmotic pressure (between 55-85 bars for seawater) imply large amounts of energy are required. Typical energy consumption ranges from 3-8 kWh/m3, depending on system size and feed water quality. The quality of the product water generated is directly related to the quality of the water source. For feed waters exceeding TDS ~ 50 g/L, the effectiveness of Reverse Osmosis (RO) as a purification technology reduces significantly. In addition, filtration techniques have other limitations, such as the need for pre-treatment and the high use of chemicals to prevent fouling and scaling. These limitations result in a high operational cost, high technical complexity and environmentally-unfriendly operations.
A new vision in water
Many technologies are inherently inefficient. They dissipate a large fraction of the energy they consume as low temperature heat. To solve this there is a strong focus on developing more efficient products. Cars, computers, TVs all now consume less energy than they did in the past. A well-known example is the development of LED lamps. While traditional lamps use about 5% of the energy to produce light, LED lamps increase their efficiency up to 25%. However, even in this case, 75% of the energy is wasted as heat. Netherland’s based Aquaver began to explore a different approach to technological innovation in 2011. In nature, for example, there is no waste. This is an approach Aquaver wanted to mimic. Aquaver started to investigate the possibility of using waste heat to power water treatment systems.
The underlying technology
The water treatment systems developed by Aquaver are based on a patented Multistage Vacuum Membrane Distillation (VMEMD) technology (process is illustrated in Figure 2). Pre-heated feed water is fed into the first stage of the processing module and flows through a channel bordered by a thin plastic foil and a hydrophobic membrane. The stage’s low pressure allows the feed water to boil at a reduced temperature, and the water vapour gently passes through the membrane. The steam subsequently condenses onto a foil to a pure distillate, delivering its heat to the next stage. Contaminants, such as salts, remain dissolved in the now slightly more concentrated feed stream. The feed water stream passes onto the next stage, where the pressure is even lower. This enables the membrane distillation process to repeat itself at a lower temperature driven entirely by the heat of condensation from the previous stage. At each stage clean water is extracted and the feed stream concentrates further. At the end of the processing module distillate and concentrate are collected in separate tanks. Energy (low temperature heat at 60-80°C) only has to be supplied to the first stage. The evaporation condensation process is repeated several times, as described in Figure 2, multiplying the thermal efficiency or Gain Output Ratio (GOR). [The Gain Output Ratio is the number of kilos of distillate produced per one kilo of steam introduced in the system. The GOR is a common measure of the thermal efficiency of a system.] Typical GOR of 3-6 can be obtained with this novel technology. The process is based on thermal separation. Thermal separation, in contrast to mechanical separation, is based on the difference of the boiling point (volatility) of the different constituents, relative to the boiling point of water. Particle size or concentration levels become irrelevant in this process. Salts, for example, which are difficult to remove through filtration, are easily removed in the thermal separation process. They have a boiling point a few orders of magnitude higher than water. Because of this, thermal separation processes can ensure that water product quality is independent of the feed water, since the product is obtained through distillation.
Products characteristics
The basic functioning of the system is described in Figure 3. Feed water is fed into the system, which is heated by low temperature heat. The system then produces clean water and a concentrate with all the contaminants. There is also a post-treatment unit which mineralises the water to make it fit for human consumption. 1. Feed water, such as seawater, is fed into the system. 2. The system is powered by low temperature heat (60-80°C). 3. The system produces clean water with consistent quality. 4. The contaminants leave the system in a brine stream (concentrate).
Clean water production
The nature of the distillation process ensures the Aquaver system delivers a high quality product independent of the quality of feed water. In order to demonstrate this, a series of application tests were conducted. An example of one of the tests is illustrated in Figure 4, where commercial synthetic seawater (Instant Ocean) at different concentration levels was treated using one of the commercial Aquaver products -a WTS-40, which delivers 60l/h of clean water. The concentration level ranged from 17 gr/L (0.5 × seawater salinity) up to 175 gr/L (~5 × seawater salinity). The system produced a consistent-quality distillate with <2 mg/L TDS for all the different salinities. [Fresh water is usually defined as < 1500 mg/L TDS.] This is around four orders of magnitude lower than the salinity of the brine. With this technology, it is possible to have a truly one-step-process for water production without a need of a pre-treatment stage. The complete set of experiments was performed with one simple 40 μm particle filter to protect the processing module.
Sustainable systems
The electricity consumption of the systems is just 2 kWh/m3, and it is basically for the pumps, which work regardless of the output volume or seawater salinity. This is a remarkable improvement in energy efficiency when compared to desalination by mechanical filtration, which usually consumes 3-8 kWh/m3. In addition to electricity, the systems operate on low temperature heat. Thus, they can be powered by a wide range of systems that produce low temperature heat, such as waste heat from diesel generators, or solar heat collectors (Figure 5).
Low maintenance
The membrane distillation modules are made of polypropylene (PP) and polytetrafluoroethylene (PTFE) membranes. Both materials are corrosion-proof plastics. In addition, the hydrophobic nature of the membranes and the low temperature operation of the system (<80°C) significantly reduce fouling and scaling, which are sources of major concern in water treatment. Just a regular simple citric acid flush fully restores the system performance. This minimises expenses for new chemicals as well as costs associated with discharge of chemical waste. All the above results in a very competitive water cost.
Conclusions
Aquaver has developed novel water treatment systems which offer the next stage in energy efficiency: advanced products powered by heat. The systems produce clean water from almost any water source using low temperature heat. This has major implications for energy consumption, because it can be driven by waste heat or by renewable sources. For desalination, pre-treatment is not required and maintenance is very low. Aquaver has developed products capable of producing from 1-100m3/day of drinking water. The products are particularly interesting for off-grid applications, such as those in remote locations.
References
1. Global analysis and assessment of sanitation and drinking water (GLAAS). World Health Organization (WHO). (2012). 2. Global threats to human water security and river biodiversity. Nature 467, 555–561 (2010).