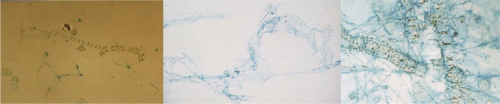
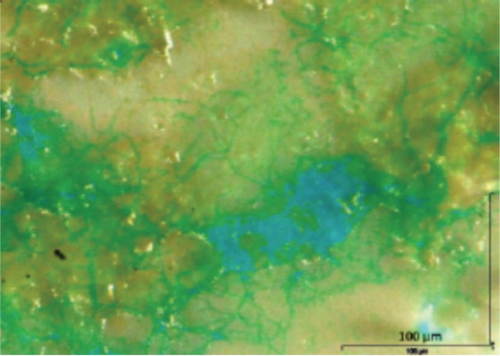
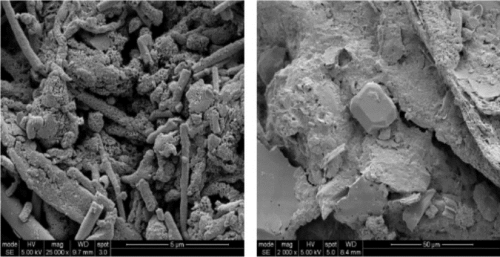
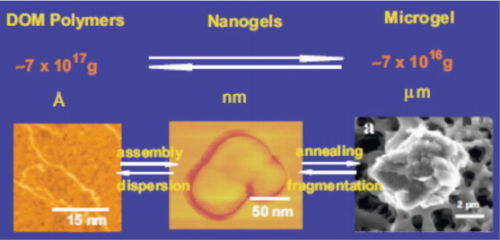
When reverse osmosis (RO) membranes were introduced, one of the first problems identified with the technology was biofouling. Today, biofouling is recognised as at least a contributing factor to more than 45% of all membrane fouling. This makes biofouling well known, ubiquitous and an almost accepted part of operating an RO system.
Biofouling degrades overall plant operations by reducing flux rates, increasing the amount of reject water, decreasing permeate quality, increasing energy consumption and ultimately causing the premature replacement of membrane elements. While chemical cleaning will improve membrane performance, rarely does the post-cleaning efficiency return to pre-cleaned levels. Therefore, each cleaning brings the element one step closer to replacement.
We are able to recognise bacteria as the main cause of biofouling through the study of the literature, service providers, operational experience and through the forensic analysis of fouled membranes. Once biofouling takes root, many other organic and inorganic materials contribute to building the fouling mass. Articles appear on a regular basis that repeat a version of the same information on biofouling: bacteria cause biofouling and they make the same observations about reducing bacteria or SDI as its treatment. This repetition reinforces the belief that biofouling is well understood and is being properly addressed by the industry.
Why is so much time, effort and money being invested by many different industry providers to reduce membrane biofouling, yet these efforts are producing only incremental improvements? One way to look at this issue is from the perspective of the basic technology platforms being used by the membrane producers and for biofouling remediation. For example, no matter what improvements are made or how much money and time are invested in a propeller-driven airplane, that technology platform will never break the sound barrier. That does not make the propeller plane obsolete, nor does it make the jet that can break the sound barrier always the better aircraft. The critical point to comprehend is that until the limitations of any technology are acknowledged, how can the correct platform be selected for the right application? The membrane industry may be essentially at its technological crossroads in terms of membrane modification or chemical water treatment techniques to make additional, significant reductions in biofouling.
Barriers blocking acceptance of breakthrough technology
Consider this question; if there were some new breakthrough technology that could significantly reduce biofouling, how would I learn about it or even be able to recognise it if I saw it? There are a number of hidden barriers standing in the way of answering this question.
• What we ‘know’ about the problem is correct.
Everybody knows that membrane fouling begins when bacteria attach to the membrane surface and other internal components of an element. But what mechanism actually causes the bacteria to attach to the membrane surface in the first place?
• When an operator does not believe there is a biofouling problem.
After trying many treatment techniques to establish their current cleaning protocol, an operator knows the biofouling treatment being used cannot be improved; therefore there is not a biofouling problem. With this belief firmly entrenched, it would seem fantastic to think that there could be a cost effective way that in some cases, extend the time between CIPs several fold.
• Reducing the Silt Density Index reduces biofouling.
Evidence proves that reducing SDI reduces biofouling. While this is true, does SDI directly measure the actual contaminant in the water that causes biofouling?
Breaking through the barriers
To break through these barriers, it is necessary to acknowledge that perhaps some of the things we think we know about membrane biofouling may not be completely true. Next, it is important to learn how to discover and implement truly new products or ideas that could be part of a solution to the problem. Finally, we must learn to recognise the difference between what are often presented as new ideas but are really just ‘new and improved’ versions of the existing technology.
Innovative work on the root cause of membrane biofouling is now being published by universities, as well as emerging from data resulting from commercial testing of new filter media. This work is generating compelling evidence that the underlying cause of membrane biofouling is not directly by the bacteria themselves but indirectly through the polysaccharides produced by the bacteria.
TEP – the real culprit
These polysaccharides or combinations of polysaccharide and other cell debris are referred to in the scientific literature as Transparent Exopolymer Particulate (TEP) or Extracellular Polymeric Substances (EPS). Some researchers would correctly argue that there is a difference between TEP and EPS, but for the purposes of this article they may reasonably be considered as being the same material.
TEP are produced by phytoplankton, bacterioplankton, microalgae and possibly higher organisms such as shellfish as protection for the organism's outer membrane surface. The polysaccharide layer is periodically regenerated, just like a layer of our skin, with the old layer being sloughed off to float free in the water as the new layer is developed.
TEP behaves like a gel, is electronegative and has a surface that is highly reactive, being up to four orders of magnitude stickier than most particulates. It ranges in size from 0.04–200μm (see Figure 1) and can be deformable enough to pass through an 8 kDa membrane.
The unique properties of TEP create the perfect agent to bond with the electropositive surface of membranes and element components to become the initial cause of biofouling. TEP fouling of a membrane surface can be clearly seen as the green and blue stains in Figure 2.
Once the membrane is contaminated with TEP it acts as an adhesive to trap viruses, bacteria and inorganic particulates that are all part of a feedwater stream. The TEP also acts as a food source for trapped bacteria to support their proliferation and generation of more TEP. This cycle of bacterial entrapment and TEP generation exacerbates the biofouling of the membrane, feed spacer and other components of the element.
Since TEP are a product of the organism's outer cell structure and other cell debris that float freely in the water, the bacteria themselves should have little effect on biofouling. Tom Berman introduced the idea of TEP being involved in biofouling as well as doing recent work showing no difference in biofouling when using feedwater containing live or inactivated bacteria. This helps to explain why disinfection of the feedwater does not eliminate biofouling since these treatments do not address the actual source of fouling.
Research has also found that TEP nanogels can assemble and disassemble themselves through a two step, reversible process. This capability allows TEP to exist in various, constantly changing forms and shapes along the dissolved-particulate size continuum. Figure 3 by Verdugo shows TEP across this size continuum from dissolved organic material to microgel particles. Verdugo's work has found that when filtered through a 0.2μm membrane the TEP is present in the filtrate as free polymers that reassemble into nanogels 5–10 hours after filtration. This feature of TEP makes it very difficult to remove using standard filtration techniques.
These characteristics explain why TEP is largely transparent to sand filters, most hollow fibre membranes and even UF membranes. Since TEP is by definition transparent, SDI is not really an effective measure of biofouling potential of feedwater. The real challenge is to measure and reduce TEP, not necessarily the SDI or the bacteria themselves, in order to reduce biofouling.
Reducing TEP reduces membrane biofouling
Many membrane producers are promoting the use of NF or UF membranes as pre-treatment to RO. This technique is rather expensive and energy intensive. Membrane pre-filters have been shown to work quite well to protect RO membranes from biofouling. Many operators have also learned from bitter experience that their use often becomes a very expensive way of transferring biofouling from the RO to the pre-filtration membrane.
Because TEP is ‘sticky’, deformable and electronegative it is perfectly suited to be effectively removed from water using media that does not rely on mechanical removal processes. Using electropositive, nonwoven filter media as a pre-filter to RO membranes is one possible RO pre-filtration tool. Unlike membranes, electroadsorptive media has a rather large pore size which presents energy savings through a very low pressure drop. The electroadsorptive nature of the media allows it to reduce the amount of TEP regardless of its size or shape in the dissolved/particulate continuum at the time of filtration. A typical pleated filter cartridge of 115mm in diameter by 1m in length will have a surface area of about 2 square metres and less than 0.2 bar pressure drop. This filter could efficiently process up to 240 litres per minute. Certain adsorptive media have been shown to remove significant amounts of TEP effectively from fresh, brackish and salt water. Figure 4 shows an electroadsorptive media.
Electroadsorptive media is an effective sub-micron polishing filter for removing or reducing second-order foulants that include endotoxins, viruses, bacteria, organic acids and colloidal metals such as iron. However, electroadsorptive media does itself need to be used with appropriate pre-filtration to avoid premature surface blinding before all the adsorptive attachment sites are utilised.
Conclusions
Biofouling was identified at the same time as the introduction of RO membranes and yet 30 years later the problem is still with us and being addressed using ‘new and improved’ versions of the same basic solutions.
No one membrane technology, system design or filter will ever provide a single solution to remediate the myriad of contaminants present in RO feedwater. When properly applied, every technology has the potential to add value as part of a complete system solution. In order to introduce breakthrough technology solutions for biofouling into mainstream use will require operators to challenge their service providers, systems designers and systems builders to identify and present them with promising new solutions.
References
Berman T. and M. Holenberg. 2005. Don't fall foul of biofilm through high TEP levels. Filtration+Separation. May 05: 30-32.
Berman T. TEP – a major challenge for water filtration, Filtration and Separation (March/April 2010).
El-Azizi, I., Edyvean, R.J.G. – University of Sheffield, UK; Study on a Novel Depth Filter (Disruptor™) for Reduction of SWRO Membrane Fouling.
U. Passow, Production of transparent exopolymer particles (TEP) by phyto and bacterioplankton, Marine Ecology Progress Series 236 (2002), pp. 1–12. Full Text via CrossRef | View Record in Scopus | Cited By in Scopus (47)
L. Villacorte et al., The fate of transparent exopolymer particles (TEP) in integrated membrane systems, Water Research 42 (2009), pp. 5039–5052. Article | PDF (3359 K) | View Record in Scopus | Cited By in Scopus (1)
P. Verdugo and P. Santschi, Polymer dynamics of DOC networks and gel formation in sea water, Deep Sea Research II 57 (2010), pp. 1486–1493. Article | PDF (764 K) | View Record in Scopus | Cited By in Scopus (2)