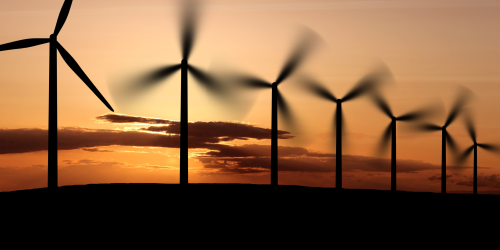
Introduction
Filtration systems are increasingly being powered by renewable energy sources. Current installations use the more developed technologies of solar and wind power as well as biogas combustion in combined heat and power (CHP) engines for this purpose. Water treatment plants are also being viewed as excellent potential locations to install wind and solar power, while other renewable sources can potentially be exploited at certain sites.In this article, we also focus on how membrane technology can be useful in anaerobic biogas generation from wastewater, and how membrane technology is used to purify raw biogas itself to permit its routine use. Similar technologies can also be used aerobically when combined with suitable sludge extraction to generate an alternative feedstock to oil-crops for biodiesel generation. We also briefly look in a wider context of a membrane filtration process used in an energy recovery scheme. Not just a renewable energy consumer, filtration technologies play an important role in generating renewable energy sources too.
Powering wastewater treatment with renewable sources
Renewable sources have been used to power a variety of everyday devices, such as roadside signs, and industrial plant, for example biogas at landfill sites to power pumps and monitoring equipment, for several years now. The wastewater treatment industry is likewise investigating the feasibility and commercial viability of renewable energy sources. In recent years, the potential for siting wind and solar power renewable energy sources at wastewater treatment plants has been investigated. These more established renewable technologies have gained favour with several operators, whilst alternative hydropower and yet other possibilities such as geothermal have been mooted as potential renewable energy sources for the sector in the academic world.
In 2005, the Atlantic Counties Utilities Authority (ACUA), New Jersey, USA, commissioned a combined solar-and-wind scheme for on-site power generation at its Atlantic City wastewater treatment works. This system features a 0.73 MW solar power system, including two roof-top and two ground level arrays with a supplementary arrays on solar car-ports. Five 1.5 MW GE wind turbines are also installed, generating up to 7.5 MW of power, sufficient for 2500 homes in combination in addition to powering the water treatment plant. This provides a good example of what is possible, and companies such as GE Zenon have installed smaller-scale membrane filtration plant powered by solar and wind power.
Renewable energy for RO desalination
One particular application in which renewable energy has found favour in wastewater treatment sectors around the world is to provide power for membrane ultrafiltration and reverse-osmosis (RO) desalination. Using membrane technology remains the most feasible and energy efficient method of desalination in a world looking to increase its capability to reliably generate potable water. With over 15000 desalination plants worldwide, the application of renewable energy to this typically energy-intensive process is worthy of particular consideration in both the developed and developing world.
As with the ACUA New Jersey plant, the use of better established wind and solar power technologies tend to be viewed as more feasible. The viability of combined solar power and desalination plants has being investigated for regions such as the Mediterranean (e.g. F. Trieb et al. Desalination 153, 39-46, 2003). A detailed feasibility study into the integration of wind energy and RO desalination conducted in USA sponsored by the National Renewable Energy Laboratory (NREL, 2005) identified the key constraints on the acceptability and adoption of such systems within the U.S.A. Identified constraints include the operability over a large power envelope, the robustness of feed-water variation, the management of often conflicting requirements, the in-situ monitoring of membrane deterioration, and reducing the overall cost of water. A particular focus of the feasibility study was on how best to integrate the operation and maintenance of the RO membranes under fluctuating power input conditions from wind turbines.
This study modelled various plant component modules, including an assumed spiral-wound polyamide membrane RO units and wind power generator, converter and energy storage modules, to investigate several possible energy source-RO configurations, with further models to evaluate the relative cost of water in each case from both seawater and brackish raw water sources. Using this systematic modelling approach the study concluded that it is feasible to generate potable water cost-effectively under configurations that are both connected to the grid (USD 0.77/m3) and in areas where grid connectivity is not possible by virtue of site remoteness (USD 1.11/m3). In both cases it is possible to model the optimum plant size. Thames Water will construct the first large-scale desalination plant in the UK over the next year at Beckton, London, with a view to operating in 2009. The Thames Gateway Water Treatment Plant (TGWTP) will supply up to 140 MLD of potable water, enough for 400,000 households, by processing estuarine water captured during the ebb tide (in order to be of lower salinity than if captured during flood tide, hence requiring less desalination). Although normally considered an expensive option, lack of local fresh surface water and limited groundwater extraction possibilities mean desalination local to London in more attractive compared with fresh water import options, although its route through the planning process has not been without controversy. By capturing low salinity water less than half of the energy required to desalinate full strength seawater is used. Output from the plant will be variable, allowing lower processing rates (to only 25 MLD) when water demand is less intensive or can be met more readily from other sources.
The treatment process at TGWTP will involve four-stage RO desalination after water flocculation and filtration pretreatment, with subsequent remineralisation and disinfection. An important commitment for this design is that it will run on 100% on renewable energy. Initially, this commitment will be met by running the plant with biodiesel to supply the estimated 6.3 MW annually. Ultimately, the objective is to use wind power to meet as much of the energy requirement as possible, either from an on-site wind farm or by installing wind power at other local utility sites and operations. Thames water currently use renewable energy to power local sewage treatment plants. At both Beckton and Crossness, sludge powered generators are used to incinerate high calorific value sludge without digestion; elsewhere, biogas is used in several CHP plants for on-site power generation.
Enhanced biofuel production using filtration systems
We have discussed the ways in which renewable energy sources are being used to power membrane filtration systems across a range of applications. The enhanced particle removal capability of membrane barrier systems can also improve the capacity to generate and manufacture renewable energy sources through the more efficient entrapment of organic colloids and particles. This organic material can subsequently undergo controlled anaerobic degradation, together with other advanced treatments, to produce methane-rich biogas and other usable fuels such as biodiesel.
In most applications, membrane filtration systems are used aerobically, removing suspended particulate matter (SPM) to a degree fit for the application purpose, including the SPM organic fraction. A variety of aeration techniques may be used, normally in conjunction with a bioreactor in order to oxidise this organic matter to reduce wastewater chemical oxygen demand (COD) to an acceptable level prior to effluent discharge. This process, though very efficient and effective, can be expensive and is power intensive (comprising up to 5% of U.K. energy use). Recently, heightened attention has been paid to using membrane filters in anaerobic treatment systems.
Anaerobic processes have been widely and effectively used in the solid waste treatment sector (e.g. landfill), and in the wastewater sector for sludge treatment. Biogas is generated under mesophilic (20 – 40°C) or thermophilic (up to 60°C) elevated temperatures, consisting of methane together with a number of impurities dependent upon the waste matter degraded. For example, in the U.K., a typical landfill waste-stream (at 62% biodegradability) can produce up to 150 m3/tonne biogas at about 60% methane concentration. Even accounting for process inefficiency and anaerobic plant power requirements, biogas can potentially form an important renewable energy source, some estimates suggesting this could be almost 2% of the U.K. demand (Waste Research Station, Cardiff University). Significant research efforts have recently investigate conditions to optimise biogas generation rates and improve methane enrichment and impurity reduction or removal in these processes, further increasing the potential of this renewable energy source.
An additional advantage of burning biogas for energy production is that the methane (with a greenhouse warming potential of 62 times that of CO2 over a 20 year time horizon) is largely converted into carbon dioxide, reducing the overall greenhouse impact. In the U.K., the use of biogas as a renewable energy source has not been well exploited. Whilst local combined heat and power (CHP) schemes and industrial CHP systems that power plant and equipment on-site are fairly widespread, and latterly small-scale farm biogas plants have been installed. the U.K. has yet to adopt large-scale centralised biogas generation facilities such as those in Denmark and elsewhere in Europe.
Anaerobic degradation processes can also be applied in wastewater treatment processes. Funding has been awarded to Professor David Stuckey (Imperial College, London) to develop a pilot plant for his submerged anaerobic membrane bioreactor system (SAMBR). The key advantages to this approach are the very low power requirements compared to aerobic treatment and the low production rates of waste reducing the subsequent sludge treatment requirements. In this process, a 0.4 μm membrane is directly submerged into the wastewater reactor tank. The physical membrane barrier retains all of the biomass and a significant fraction of large (high molecular weight) soluble organic compounds. The anaerobic micro-organism population completes biogas generation (including methanogenesis) from the retained matter within a typical time-scale of 3 hours. COD reduction in the permeate is typically better than 99%, the high efficiency largely due to the effectiveness of the membrane in organic matter retention. The biogas generated scours the membrane surface to reduce fouling, improve permeation rates and reduce the trans-membrane pressure.
Whilst anaerobic wastewater treatment is not a new idea (for example, Veolia market anaerobic treatment plant for the treatment of wastewater from the food & drink, paper & pulp and chemical sectors), the SAMBR may offer the potential for completely changing the approach to domestic and industrial wastewater treatment whilst generating significant quantities of biogas which, if properly purified and harnessed, could form an important national source of renewable energy.
Membrane technology is important in biogas purification. With relatively cheap manufacturing costs and a wide range of highly specific membrane surfaces now available, biogas purification can readily result in a methane purity in excess of 96%. There are two main approaches to biogas membrane clean-up, namely gas-liquid absorption (which works at approximately atmospheric pressure) and high pressure gas-gas separation. In the former case, biogas molecules flowing counter-current to an absorptive liquid diffuse through a microporous hydrophobic membrane. Typical absorbing liquids include sodium hydroxide for hydrogen sulphide removal (typically reducing 2% raw biogas H2S concentrations to less than 250 ppm) and amine solution for CO2 reduction (from about 43% to less than 4%); in both cases it is possible to reuse or regenerate the absorbing liquid. For gas phase membrane purification, biogas which has been pre-treated over activated carbon to remove halogenated compounds, some hydrogen sulphide and oily vapours and passed through a particle filter, can next be passed through a series of membrane filters. These specifically remove polar molecules including water vapour, H2S and CO2. Gas-gas purification occurs at pressures around 36 bar; having pressurised the influent biogas, purified product can readily be stored in steel cylinders at a range of pressures up to 250 bar.
Membrane technology can also play a role in biodiesel generation. With the current concern that the drive for biofuel production comes with detrimental effects on world food supplies, alternative biofuel production mechanisms are of interest. Normally, biodiesel is produced from the trans-esterification (the conversion of one ester to another) of oils from oily plants such as rapeseed or sunflower with a methoxide catalyst to produce separate fuel oil and a glycerol by-product. However, it is possible to extract the lipid fraction of municipal sewage sludge as an alternative feedstock for biodiesel generation. Research into lipid extraction optimisation has been published (S. Dufreche et al., J. Amer Oil Chem Soc, 84, 181-7, 2007). Here lipid extraction of dried sludge using a polar mixed (methanol/acetone/n-hexane) solvent, followed by in situ trans-esterification resulted in a biodiesel yield of 6.23%. Using conventional aerobic membrane bioreactor systems, sludge generated can potentially be used for biofuel manufacture.
Conclusions
Renewable energy sources are increasingly being used to power many industrial plant items including filtration equipment at wastewater treatment plants. Indeed, many plant sites offer valuable potential locations for the siting of wind, solar and other renewable sources. Most installations with integrated renewable energy currently use well developed technologies, namely CHP (biogas), wind power and solar energy.
Special focus has been placed on integrating renewable energy with RO desalination in the search to provide sustainable potable water supply together with sustainable power for such systems. Systematic modelling in the U.S.A. Has demonstrated the feasibility of safely integrating variable wind power into RO systems, both when connections to the grid are possible and when they are not. Ambitious RO desalination capacity powered entirely using renewable energy is currently planned in the U.K. at the Thames Gateway Water Treatment Plant.
Membrane filtration technology is proving valuable in the generation of renewable energy in itself. Used in anaerobic systems, the excellent particle removal capabilities of membrane filtration allow excellent COD removal from the permeate whilst retained particles can generate significant quantities of biogas. Membrane technology can further be used in biogas clean-up to remove carbon dioxide, hydrogen sulphide and moisture to purify the biogas to 96% methane.
These, and other innovative inter-relationships between membrane and other filtration applications and renewable energy, both as a power source and renewable source generator, are set to strengthen as technologies develop, and proven feasibility is more widely accepted and adopted.