Our world is heating up at an unsustainable rate. We are already at 1.2°C/2.2°F above pre-industrial levels and moving closer to the 1.5°C/2.7°F threshold that scientists warn we must not surpass. If the earth becomes 2°C/3.6°F hotter, an increase of just half a degree Celsius will severely damage lives, livelihoods and ecosystems.
To prevent worsening climate change and its devastating effects, the Paris Agreement and Glasgow Climate Pact have set regulations to reduce carbon emissions and reach net zero by 2050, with a 50% reduction by 2030. To achieve these goals finance and technology must align to create an infrastructure that will enable decarbonisation across every sector.
Finance generates progress
Global investment in energy transition is increasing year-on-year, but many experts believe more needs to be done. Analysts from BloombergNEF have forecast that investment in infrastructure and energy transition technologies will need to triple to around US$2.1 trillion per year between 2022–2025 and then to double again, to approximately US$4.2 trillion between 2026 and 2030 to reach net zero goals.
Electric transport has seen the greatest rise in financial backing over the past decade, with upturns also in renewables, electrified heat, hydrogen production, energy storage and other sustainable practices.
Accelerating the EV revolution with battery purity
Electric vehicles are predicted to account for more than 40% of new car sales by 2040, yet there are limiting factors to the EV revolution. One main consideration is the performance of lithium-ion batteries as the materials used in their manufacture directly affect the ultimate capabilities of the vehicle.
The three components of lithium-ion batteries that are most at risk of contamination during manufacture are separators, cathode active materials, and liquid electrolyte. The ‘cleanliness’ of each individual component – often at a microscopic level – has a significant impact. As components get exponentially smaller and more sensitive to contamination, robust quality control becomes increasingly difficult.
During the separation manufacturing process, each aspect – including process water, paraffin liquid, plasticiser, polymers and protection liquid – has a different requirement. In cathode manufacture there are dozens of processing lines that need an array of cleanliness and quality controls, from oxygen and nitrogen to solvents and pure/mixed solutions. With liquid electrolyte, the high degree of acidity of the electrolyte, which requires EFTE-coated stainless-steel vessels, and the high cleanliness levels require very fine particulate removal ratings (from 0.45µm to 2µm).
Realizing the potential of green hydrogen
Green hydrogen is formed from water split by electricity generated from renewable sources and is key to global decarbonisation. During electrolysis, hydrogen is produced at the cathode and oxygen at the anode with an electrolyte present in between the electrodes.
Hydrogen stream has to be processed to remove solid, liquid and gaseous contaminants. Typically, concentrations of between 2,000-6,000 parts per million of oxygen and more than 2,000 ppm of water are seen in hydrogen using commercial alkaline electrolysis. Stringent regulations mean that the maximum concentration allowed for fuel cell vehicles is 5 ppm of each, so the gas has to be decontaminated.
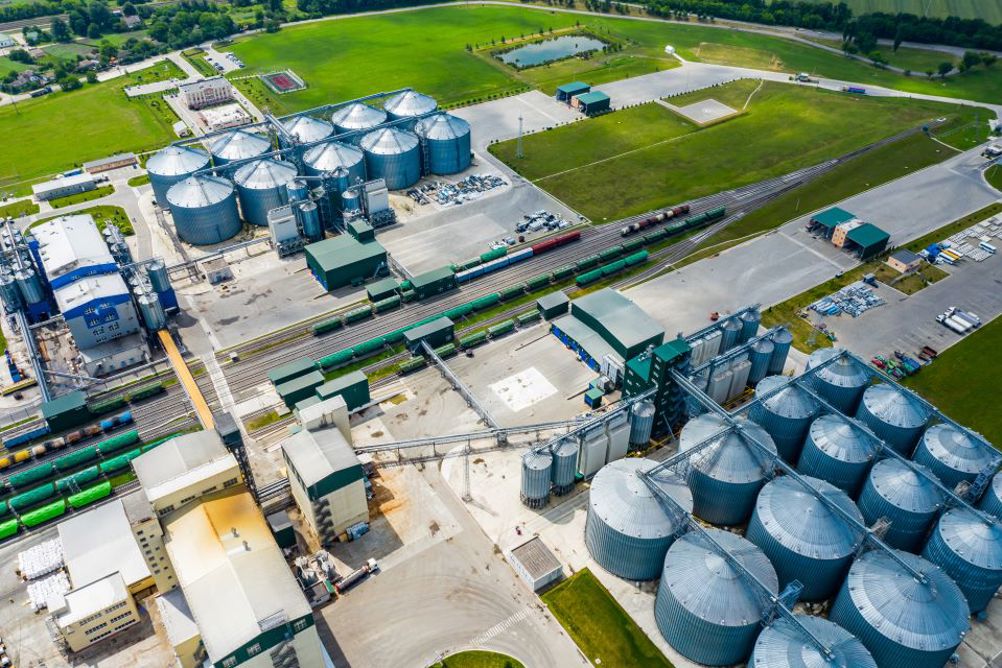
Powering the future with advanced biofuels
Demand for biofuels is expected to increase 28% from 2021 to 2026, according to the International Energy Agency. ‘Advanced’ biofuels are produced from wood chippings and non-edible plant material, residential waste such as cooking oil, industrial and commercial waste including beef tallow, and algae. Their differing molecular composition leads to varying levels of solid particulates, water content, gels and waxes, as well as variable particle size, density and viscosity. They are pre-treated to turn them into a consistent form prior to refining, which may include physical processing (chipping or milling), chemical (addition of acid or alkali) or biological (use of microbes or enzymes) techniques.
Conversion of biomass into biofuels can be done in existing refineries, with ‘co-processing’ optimising capacity. Most co-processing happens in hydrotreaters, hydrocrackers or fluid catalytic crackers. These catalytic processes remove sulphur, oxygen, nitrogen and metals.
Propelling the circular economy
Twice as much plastic is now produced compared to two decades ago, yet only 9% is successfully recycled. As recycling targets become more aggressive, capabilities for converting waste into useable products must be increased.
We must also look at what else we can recycle – for instance, old vehicle tyres can be turned into fuel through pyrolysis. This is the thermal decomposition of materials at temperatures of 400–600°C/752–1112°F in an oxygen-free environment. The breakdown of polymers to monomers uses a chemical catalyst and leads to a composition of 80% oil, 15% gas and 5% carbon black (ash). The results can be used for the same applications as fossil fuels – heat, transportation, and the production of electricity. In this way, the circulatory loop is closed: from crude oil and natural gas to plastics and other products, and then back to fuel again.
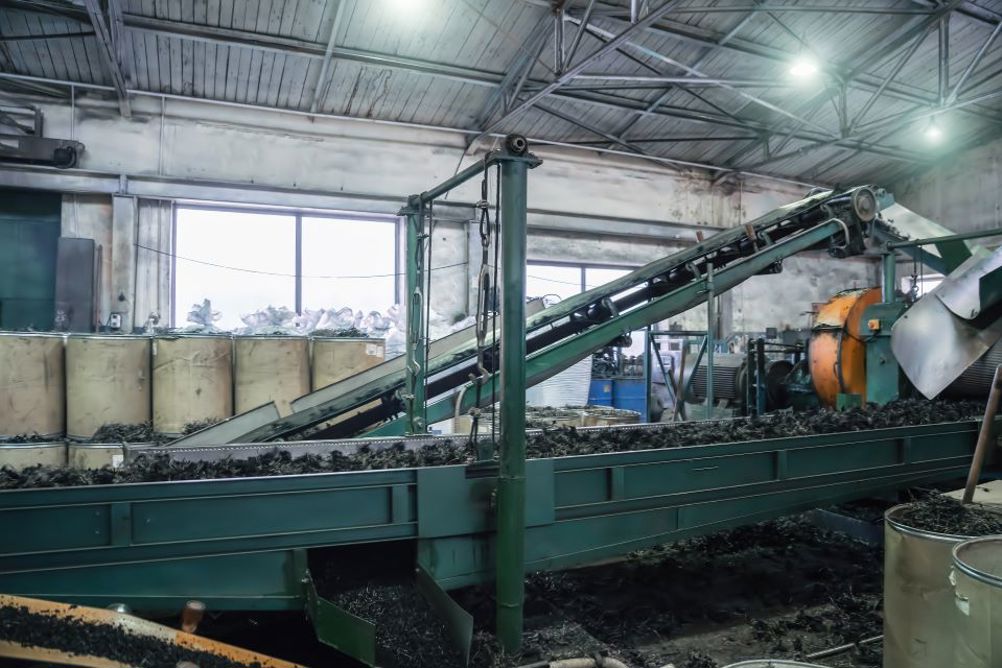
Reducing emissions with carbon capture
Although the transition to green energy is making progress, we still need to deal with the emissions produced by an ongoing use of fossil fuels. Carbon capture, utilisation, and storage (CCUS) is key. CCUS technologies remove carbon dioxide from fuel combustion and industrial processes and transport the gas either to be used to create products – such as plastics, concrete, or biofuel – or stored deep underground in saline aquifers or depleted oil and gas reservoirs
There are several methods for capturing the CO2 but the most widely used is chemical absorption. Flue gas containing carbon dioxide and nitrogen is passed through an amine solution which binds with the CO2. The solution is heated to regenerate the amine and release the CO2. Challenges include the need for large absorber vessels which can be costly and impact ROI.
Carbon capture can also be done via membrane separation, cryogenic separation, molecular sieves, and chemical looping.
The role of filtration technology
The transition away from a reliance on fossil fuels is complex, but practical options can be leveraged to make a greener future possible. From materials processing and polymer decomposition to product creation and use, there are many procedures for which filtration is not only beneficial but vital. In protecting critical operating assets, improving product quality, safeguarding health, and minimising emissions and waste, there are solutions that can be tailored to customer requirements.
Collaboration leads to success
As industry and society move to decarbonize, there is an opportunity to take advantage of existing systems and explore emerging technologies. If we innovate, collaborate, and expand our view of what we think is possible, we can make real progress on the path to using our planet’s resources more sustainably and driving the transition to a greener energy future.
The choices we make today will mean the difference between living in harmony with the environment tomorrow or suffering further from climate change. Filtration technology and separation science are key to enabling this transition.