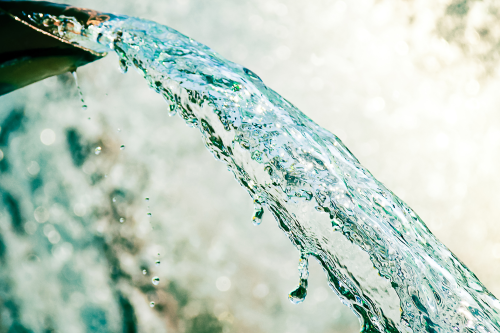
Ultraviolet germicidal irradiation is a disinfection method that uses short-wavelength ultraviolet light to kill or inactivate microorganisms by destroying nucleic acids and disrupting their DNA, leaving them unable to perform vital cellular functions. The global market for ultraviolet (UV) disinfection equipment was valued at $790 million in 2010 and is expected to $2.1 billion by 2018.
UV light for disinfection has been used for more than 30 years, but the technology has expanded from a niche market to hold a significant share of the disinfection equipment market only in the last decade. Water and wastewater treatment equipment is the largest and one of the fastest growing sub-segments of this market.
Pars Mukish, a consultant with Yole Development in Lyon, points out that the production of mercury vapour lamps is set to decline due to recent international agreements that limit the production, use, and trade of mercury. These include the Minimata Convention on Mercury, which so far has 128 signatories including Japan, China and the US. The gap left by the withdrawal of mercury vapour lamps will need to be filled with a new source of UV light and it is clear that the product waiting in the wings for this role are Ultraviolet Light Emitting Diodes (UV-LEDs).
Dentist using dental UV curing light led laser. (Image courtesty of sima/Shutterstock).
LEDs on the rise
The humble light-emitting diode (LED) has come a long way since its origins in 1960s America. There, in the heady days of the semiconductor revolution, researchers at labs including RCA, General Electric, Bell and Texas Instruments worked to develop modern LEDs. The first of these could only emit infrared light, but doping the materials with additives soon led to LEDs that emitted visible light. Some of us still remember the resulting red-on-black displays on our Texas Instruments calculators and Casio watches.
Since then, the light-emitting capabilities of LEDs have crawled inexorably up the frequency spectrum, through the visible range finally entering the ultraviolet (UV) realm with wavelengths shorter than 400 nm. Work began on developing UV LEDs in the late 1990s and within a few years the first products became commercially available, with the wavelength being driven down ever since from near-ultraviolet (NUV) (approximately 300– 400 nm) into deep-ultraviolet (DUV) (approximately 200–300 nm). The optimum range for germicidal irradiation is between 200 and 300 nm. Hence UV LEDs are now able to fulfil the germicidal irradiation tasks that had been the province of mercury vapour lamps. In the past few years, DUV LEDs have finally become commercially available cheaply and in large quantities.
The Japanese company Nitride Semiconductors developed the world's first UV LED in 2000, but other companies in Japan and elsewhere are also now getting in on the act. The market for these devices grew from $20 million in 2008 to $90 million in 2014.
Yoshihiko Muramoto, Nitride's president and chief executive officer, told Filtration and Separation Magazine that the exponential growth in the applications for UV LEDs has largely been led by improvements in crystal growth, chip-processing and packaging technologies. As a result, says Muramoto, UV LEDs have come far in the past decade. Their efficiencies have now reached 60% at a wavelength of 405 nm, 50% at 385 nm and 30% at 365 nm. In addition, the latest models emit light with a power of up to 24 W, which is more than a hundredfold increase on what was possible 10 years ago. At the same time, costs have fallen as a result of mass production, making them more affordable.
On the right wavelength
The scientific journey to reach this point has not been easy, even though a UV LED works on the same principles as any other LED (see ‘How to make an LED’). At its most basic, a voltage applied across a p–n junction diode causes light to be emitted as electrons recombine with electron holes, with the electronic energy levels – and hence the energy of emitted photons – depending on the material and doping choices. The wavelength of a gallium–nitride LED is controlled by adding the dopants indium and aluminium in different ratios.
How to make an LED
In a light-emitting diode (LED), electrical energy is converted into light energy in the form of photons that are emitted from the device. Typically they emit a narrow bandwidth of wavelengths from infrared to ultraviolet. They can also be constructed to emit laser light.
To make an LED, an electron-rich semiconductor and an electron-poor semiconductor are joined to form a p–n junction. This is a junction between two types of semiconductor material that have different proportions of electrons to electron-accepting holes. Applying a voltage across this junction causes electrons to move energy levels and produce photons – for example when electrons ‘fall’ into empty holes in the p-layer of the p–n junction. This happens in any diode – for example, in a standard silicon diode; however, in this case the frequencies of the emitted photons are so low that they are in the infrared part of the spectrum and invisible to the human eye.
This explains why doping the p–n junction is crucial to the frequency of light that is produced. Normal diodes, which are used for detection or power rectification, are made from either germanium or silicon. LEDs, in contrast, are made from exotic semiconductor compounds such as gallium–arsenide (GaAs), gallium–phosphide (GaP), silicon–carbide (SiC) or gallium–indium–nitride (GaInN), all mixed together at different ratios to produce light with a distinct wavelength.
More indium results in a shift to longer wavelengths, and more aluminium results in a shift to shorter wavelengths. So, while a conventional blue LED is made primarily of indium–gallium–nitride, a DUV LED is made primarily of aluminium–gallium–nitride.
Increasing the aluminium-to-indium doping ratio to achieve shorter wavelengths, however, has the downside of decreasing efficiency. As Muramoto explains, making the emission wavelengths of the LED shorter than 400 nm means having to dope aluminium in the active layer of the p–n junction. “But free electrons of aluminium are difficult to release because of their strong attraction to the atomic nucleus, so we need high temperatures of around 1200 °C to grow the UV LED wafer,” he adds. The problem is that these temperatures cause a problem for the dopant layer. “It is very hard to control the flow of gas accurately and achieve a uniform layer of the dopant on the sapphire substrate,” says Muramoto. To get around this problem for DUV LED wafers, a buffer layer of aluminium–nitride is first deposited on the sapphire substrate. So complicated is the physics behind the manufacture of DUV LEDs that Japanese manufacturer Nikkiso, which has been developing these devices since 2006, enlisted the help of Isamu Akasaki of Meijo University and Hiroshi Amano of Nagoya University, who went on to share the 2014 Nobel Prize for Physics for their work on blue LEDs with Shuji Nakamura of the University of California, Santa Barbara. Under their guidance, the firm modified its mass-production technologies and began selling samples of DUV LEDs in 2012.
Mercury vapour lamps are used in many technologies, and it is not only international agreements that make their replacement with UV LEDs inevitable. The simple fact is that UV LEDs have many advantages over mercury lamps: they are more efficient, last longer and emit light at a more constant intensity. It is also easy to control their temperature and heat.
Newborn baby with neonatal jaundice under blue UV light for phototheraphy. (Image courtesy of Paul Hakimata Photography/Shutterstock).
UVs in practice
As well as the NUV/DUV differentiation, the UV range has traditionally been divided into the three categories UV-A, UV-B and UV-C. Applications in the UV-A range (315–400 nm) include identifying counterfeit currency and curing resin, which involves the cross-polymerization of a UV-photosensitive material such as an ink, adhesive or coating.
Dentists, for example, use UV lamps to rapidly harden the UV-sensitive resin of tooth fillings. The intensities of 1–10 W cm–2 required for some types of resin curing have already been reached by LEDs in the NUV range, but power isn't everything. Another factor to consider is the emission spectrum of the light source being used. UV LEDs have a much narrower spectrum than mercury-vapour lamps, and it has been found that, for example, a 365 nm UV LED did not provide the precise wavelength needed to initiate curing.
Shorter UV-B wavelengths (280–315 nm) are used in phototherapy, for example to treat jaundiced babies to oxidize the liver pigment bilirubin. In the original version of this therapy, the baby is laid under a mercury vapour lamp with its eyes protected by goggles, far enough from the lamp so as not to overheat.
In adults, meanwhile, UV phototherapy helps in the treatment of psoriasis and other skin conditions. Psoriasis is a persistent and chronic skin disease that tends to be genetically inherited. The effects of psoriasis can range from a small, localized area to the entire body. With conventional mercury-vapour lamps, improvement can be seen in as little as three weeks, with maintenance therapy thereafter. LEDs will deliver the same benefits in a more convenient and efficient way.
UV light sources are also fundamental tools for forensic investigation. At crime scenes, fluorescein (a common fluorescent dye) is sprayed onto surfaces to reveal human DNA evidence such as blood, semen, skin oils and amino acids, the fundamental building blocks of proteins. UV illumination is also used by the police to discover former wounds, bite marks and bruises for six to nine months after the injury was inflicted – the kind of evidence that could prove pivotal in a court case. UV-LED-based devices will be more portable and powerful than current alternatives.
The shortest wavelengths of UV are in the UV-C range (100–280 nm). This type of UV can sterilize water, air and surfaces by breaking up the nucleic acids – including DNA and RNA – of micro-organisms, so preventing them from reproducing.
Nucleic acids readily absorb UV radiation, especially in the 240–290 nm range. The UV absorption in DNA peaks at around 260 nm, which is the range that has just been accessed by advancements in DUV LEDs. As LEDs take over from mercury lamps, costs will come down and efficiencies soar, not least because the LEDs will be cooled as the water being sterilized passes over them. The city of New York has recently installed a UV-based sterilization system based on mercury vapour lamps and it is likely that in the future other cities will install such systems based instead on LEDs.
It is clear that UV LEDs are an emerging technology with a variety of applications, not the least of which is germicidal irradiation.
Author details: Dr Richard Corfield, Science Writer and Broadcaster, Mobile: +44(0) 7940711792, www.richardcorfield.org