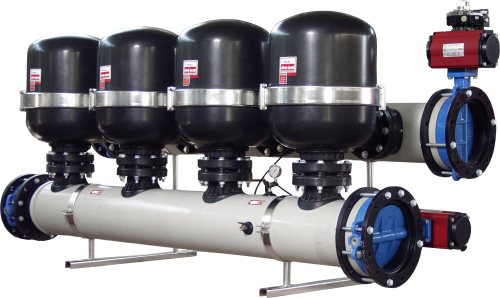
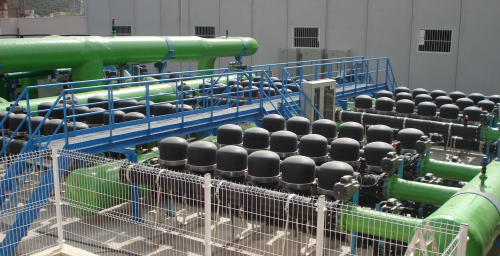
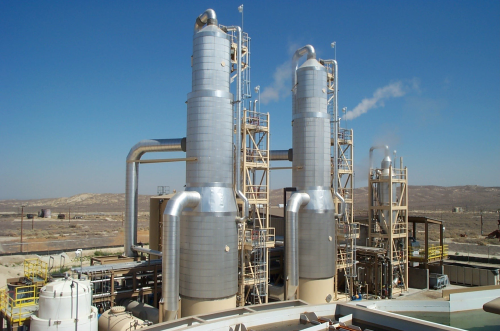
Desalination technology
Thermal desalination processes can be applied where waste heat can be used, such as on ships and offshore applications. However, due to reductions in the capital cost of membranes, and design optimisations resulting in lower operational costs, seawater reverse osmosis (SWRO) desalination systems are becoming the dominant force in large scale desalination projects.
To explain briefly how RO works we start with osmosis, a natural process involving water flow across an ideal semi-permeable membrane. Water passes through the membrane at a faster rate than dissolved solids. The difference of passage rate results in the separation of solids from water. The direction of water flow is determined by the chemical potential: a function of pressure, temperature and dissolved solids concentration.
When pure water is in contact with both sides of the membrane there is no flow because the chemical potential is equal on both sides of the membrane. If salt is added to one side of the membrane, the chemical potential of the resulting solution is reduced and osmotic flow from the pure water across the membrane occurs until equilibrium is reached. Equilibrium results when the hydrostatic pressure differential resulting from volume changes on both sides of the membrane is equal to the osmotic pressure of the salt solution.
If we apply an external pressure to the salt solution side of the membrane greater than the osmotic pressure, we raise the chemical potential of the water and cause solvent flow in the reverse direction to the pure water side. This is RO, a cross-flow membrane separation process providing a level of filtration down to ionic levels for the removal of dissolved salts. Permeate is produced from the membrane with the majority of the dissolved content of the feed. It is the treatment and/or minimization of this concentrate flow that we are concerned with in this article.
The fact that water and salt have different mass transfer rates through a given membrane results in the phenomenon of salt rejection. No membrane is ideal in the sense that it absolutely rejects salt, but in municipal desalination, membranes with less than 95% salt rejection are used so that permeate produced has a sufficiently low salinity to meet drinking water specifications.
To achieve economic levels of permeate production from seawater, the NDP needs to be in the range 80 to 120 bar, and the membrane salt rejection needs to be maximized. This means that power consumption becomes the primary component of the overall operating cost. To increase recovery and thereby reduce the concentrate volumes, NDP and membrane area increase, thus increasing capital cost. Hence, there is a balance for the process designer to strike, and considering disposal and beneficial use options for concentrate needs to be factored into design equations.
SWRO desalination systems typically operate at 50% recovery thereby doubling the concentration of salts from the seawater (approximately 3.5%) to the concentrated stream (7%). This concentrate also contains chemicals such as antiscalants used to prevent scaling within the RO membranes, along with other chemicals used in pre-treatment processes. Inland desalination systems using brackish water RO (BWRO) operate at higher recoveries, producing smaller volumes of concentrate but still with high levels of concentrated salts. It is the difficult management of these concentrated brine solutions from SWRO and BWRO systems that we will now discuss.
|
Concentrate management options
Mike Mickley, a consultant at Mickley and Associates in the US, has considerable experience in concentrate management. He said that, currently, nearly all municipal desalination concentrate sources in the US are eventually either directly disposed of, or they are disposed of after beneficial use of the concentrate. However, he said, “Beneficial uses of concentrate are rarely available, are mostly unproven, and many times do not represent a final fate solution. For example, you can use concentrate at a fish farm but after use of the concentrate you now have a concentrate with added organic waste to deal with.”
Following extensive research (including more than 98% of the municipal desalination situations in the US),
Mickley identified six options for the disposal of concentrate that currently account for nearly all situations:
• Surface water discharge (to sea or inland water course).
• Discharge to sewer (for subsequent treatment by others).
• Subsurface injection (for disposal).
• Evaporation ponds.
• Land application (irrigation or percolation pond/rapid infiltration basin).
• Landfill (for solids).
A new beneficial use of a proportion of desalination concentrate is the deployment of Amiad-Arkal’s polymeric (polypropylene or polyamide) corrosion-resistant and self-cleaning disc filter systems (Figure 1). These systems can be used as pre-filtration prior to ultrafiltration (UF) and microfiltration (MF) membranes; the primary pretreatment processes in SWRO and BWRO desalination plants. The self-cleaning technology of the disc filters is sometimes powered from the pressurized energy in the concentrate. The higher salinity of the brine also disinfects the backwashed discs (the active filtration media), as a part of the regular backwash process. A typical Amiad-Arkal disc filter system in a seawater application is depicted in Figure 2.
High recovery processing
By optimizing RO system design to maximize system recovery it is possible to minimise the volume of concentrate – this is referred to as ‘high recovery processing.’ Such an approach is more common on non-municipal inland BWRO desalination applications where disposal options for the concentrate are limited due to potential environmental impact and legislative requirements relating to the control of water pollution. Waste volume minimization has several benefits including maximizing the amount of water resource utilized and providing a feasible solution where one might not be possible otherwise.
However, Mickley says that high recovery processing does not necessarily solve the concentrate management problem. Volume reduction results in a more concentrated concentrate or mixed solids, both with minimal options for beneficial use. Mickley added, “The higher salinity (and higher concentrations of all constituents) in most cases make discharge to surface water, sewer, or land less likely. Deep well injection and evaporation ponds are still options in theory. Both, however, are very location or climate dependent. The higher salinity of the concentrate can result in a greater incompatibility with aquifer water in the case of deep well injection. While landfill may be an option, costs of land filling of solids can be high.”
Zero liquid discharge
Zero liquid discharge (ZLD) can be thought of as a subset of high recovery processing where no liquid leaves the plant boundary. ZLD systems can comprise multiple step membrane systems with some processes including thermal evaporation technology. In ZLD systems, the final concentrate is either treated by a crystallizer or sent to evaporation ponds within the plant boundary. Alternatively, for small volumes a spray dryer could be employed.
Volume reduction (including ZLD) is expensive and, according to Mickley’s research in the US, is currently too expensive for municipal applications, with the equipment and processing costs associated with volume reduction (high recovery processing – including ZLD processing) being high.
High recovery processing is widely used in non-municipal industries though, where the high costs (capital and operating) can be afforded, or need to be afforded to meet environmental constraints. Mickley commented, “In the United States high recovery is not a technical problem but a cost problem – something that has been well known in other industries. In the municipal sector, the focus is more on the cost reduction of high recovery processing.”
One new application of an existing technology that could effectively be applied as a lower cost option for the treatment of low salinity desalination concentrate is electrodialysis (ED). A project has been running at IWVA (the Intermunicipal Water Company of the Veurne Region, West-Flanders, Belgium) since July 2002 whereby UF followed by RO is employed to treat secondary effluent from a wastewater treatment plant. The treated water is then used for subsurface injection and groundwater recharge. Further wastewater treatment development work at this site has been managed by Bart Van der Bruggen, from the Laboratory of Applied Physical Chemistry and Environmental Technology at Katholieke University of Leuven and Boudewijn Meesschaert from the Katholieke Hogeschool Brugge-Oostende.
To minimize concentrate volume and improve overall system recovery, a pilot ED system was constructed and installed on the treatment site in 2008 by the Belgian company Innovation Engineering and Construction. To evaluate the economics of the proposed ED process for treating the RO concentrate, the pilot ED system was operated under various experimental conditions in three different modes: batch, feed-and-bleed and continuous. Due to the high scaling potential in the RO concentrate (1,300-1,600 mg/l as carbonates), decarbonation by adding hydrochloric acid, and ozonation were used to improve the biodegradability of the ED concentrate, hence reducing the potential of organic compounds accumulating in the recirculation system.
Van der Bruggen said, “The results demonstrated that the ED process can treat the RO concentrate consistently and reliably to improve the overall water recovery to around 95%.”
Other new desalination technologies such as membrane distillation and forward osmosis are being developed due to the driver of high recovery processing cost reduction, along with energy recovery and energy reducing measures in the membrane system and downstream processes.
Salt recovery
The selective recovery of various commercial grade salts from desalination concentrate is possible, providing a revenue stream from selling the products, reducing the need for mining and the associated environmental impact, and reducing waste volumes.
Frans Durieux from GE Power and Water says the market for membrane based desalination is consistently being underestimated. He said, “The concentrate issue is more and more pressing. We are aware of the problem and have technologies to support a solution, transferring the concentrate to valuable products like kitchen salt, magnesium hydroxide, hypochlorite and other valuable products.”
William Miller, from the Tropical Agricultural Research and Higher Education Centre in Costa Rica, known by its Spanish acronym CATIE, has recently concluded that treating even a small fraction of desalination concentrate could generate revenue for desalination plants. Carbon credits could be obtained from using carbon dioxide to produce baking soda (sodium bicarbonate) which can then be heated to produce soda ash (sodium carbonate) used for glass making, and producing ammonium chloride for use as a fertilizer in local agriculture. Also, if an ammonia plant exists in close proximity or could be collocated, then ammonia and urea fertilizer could also be produced.
Various companies like GE Power and Water and Geo-Processors Pty Limited of Sydney Australia can provide ZLD technology and technologies to extract various salts from waste concentrate.
Durieux said, “The key to a good solution is the process design. We have the benefit of being able to use our core technologies like UF, NF, SWRO, BWRO and HERO as base blocks for the desalination, and our proprietary evaporation and crystallization technologies for the concentrate treatment.” An example of a large scale salt factory built by GE is shown in Figure 3. The plant depicted produces 1 million tonnes of salt per year.
Energy efficiency
Durieux added, “The focus of our innovations have been on various ways to decrease the required energy for the processes, and the use of low grade energy like low pressure steam available in power plants. And whereas earlier generation evaporators were made out of solid titanium, we now only have to use a layer of a few atoms of this precious metal in order to protect the reactors from corrosion.”
These thermal evaporators produce vapour from boiling desalination concentrate under a vacuum. The vapour is compressed and recirculated until almost complete evaporation of the concentrate is achieved. Steam from boilers or other waste heat sources feeds the evaporators, and can be transferred from one evaporator to the next to increase energy efficiency in a multiple effect system. The technology reduces environmental impact and helps ZLD to be achieved. Crystallizers can also be used, recovering specific salts and converting the remaining waste to purified water for reuse on site and solids that are suitable for landfill disposal.
Other lower technology solar solutions are available in countries with strong solar radiation and low rainfall. Here, evaporation ponds can be used, consisting of a series of excavated hollows in the ground where desalination concentrate is left to evaporate. Additionally, in these hot countries, the ponds can be used as a source of renewable energy, with heat from the sun warming the desalination concentrate prior to treatment by thermal evaporators. Various technologies are then employed to purify the crystallized salt. Also, the collocation of desalination facilities near power plants can provide the energy required for concentrate treatment through the utilization of waste heat.
Conclusions
The volumes of concentrate being discharged from desalination systems is increasing year on year, and this trend will increase as weather patterns change due to the effects of global climate change. We have seen above that various management options currently exist for disposal or beneficial uses of desalination concentrate and that new approaches and technologies are emerging to meet the challenges.
So what does the future have in store for technologies that reduce the volume of desalination concentrate? It is likely that growth will be faster in non-municipal applications but also that, in the push towards a more sustainable approach, the benefits of waste volume reduction will be realized in terms of the reduced requirement for feed supply, and the beneficial use of concentrate as a liquid resource on-site or as a source of commercial salt products. Hence, out of the problem of concentrate disposal we are likely to increasingly see valuable resources being extracted, developed and exploited from the ‘waste’ stream, especially as energy efficiency measures are introduced.